Mesothelial Tissue: The Crucial Role of Mesothelial Cells in Development and Disease
What is the significance of mesothelial tissue in organ development. How do mesothelial cells contribute to disease processes. Why is understanding mesothelial to mesenchyme transition important for medical research. What are the key functions of mesothelial cells in the body.
The Fundamental Structure and Function of Mesothelial Cells
Mesothelial cells form a single layer of specialized epithelial cells that line the body’s serous cavities and internal organs. These cells play a crucial role in maintaining the integrity and function of various organ systems. But what exactly makes mesothelial cells so unique?
Mesothelial cells possess a distinct structure that allows them to perform their specialized functions. They are characterized by a flattened, squamous morphology with microvilli on their apical surface. This structure enables them to create a smooth, non-adhesive surface that facilitates the movement of organs within body cavities.
- Produce lubricating fluid to reduce friction
- Form a protective barrier against infections and injury
- Participate in fluid and solute transport
- Contribute to tissue repair and regeneration
Research by Mutsaers (2002) has shown that mesothelial cells are not just passive lining cells, but actively contribute to various physiological processes. They secrete glycosaminoglycans and surfactant-like substances, which help maintain the non-adhesive nature of serosal surfaces and facilitate organ movement.
Mesothelial Tissue Distribution and Regional Differences
Mesothelial tissue is found throughout the body, lining major cavities and organs. However, the characteristics of mesothelial cells can vary depending on their location. How do these regional differences manifest, and what implications do they have for organ function?
A study by Wang (1974) demonstrated significant regional differences in pleural mesothelial cells in rabbits. These differences were observed in cell shape, size, and ultrastructure, suggesting that mesothelial cells may have specialized functions depending on their location.
Key Areas of Mesothelial Tissue Distribution:
- Pleura (lining the lungs and chest cavity)
- Pericardium (surrounding the heart)
- Peritoneum (lining the abdominal cavity and covering abdominal organs)
- Tunica vaginalis (covering the testes)
Ishihara et al. (1980) conducted a detailed examination of the histologic and ultrastructural features of normal human parietal pericardium. Their findings revealed that pericardial mesothelial cells possess unique characteristics that contribute to the specialized function of the pericardium in protecting and supporting the heart.
The Developmental Origins of Mesothelial Cells
Understanding the embryonic origins of mesothelial cells is crucial for comprehending their role in organ development and potential involvement in pathological processes. From which embryonic layers do mesothelial cells arise, and how does this influence their function?
Mesothelial cells originate from the mesoderm, one of the three primary germ layers formed during early embryonic development. This mesodermal origin is significant because it allows mesothelial cells to retain the capacity for differentiation into various cell types, a property that becomes particularly important in organ development and tissue repair.
Moore, Persaud, and Torchia (2015) provide a comprehensive overview of mesothelial cell development in their embryology textbook. They describe how the mesothelium forms as a result of the cavitation process, which creates the body’s major cavities lined by mesothelial tissue.
Key Stages in Mesothelial Cell Development:
- Formation of the intraembryonic coelom
- Differentiation of coelomic epithelium into mesothelium
- Specialization of mesothelial cells based on their location
- Establishment of mesothelial-specific gene expression patterns
Mesothelial to Mesenchyme Transition: A Critical Developmental Process
The mesothelial to mesenchyme transition (MMT) is a fascinating process that plays a pivotal role in organ development. How does this transition occur, and what are its implications for organ formation and function?
MMT is a process whereby mesothelial cells lose their epithelial characteristics and acquire mesenchymal properties. This transition allows mesothelial cells to migrate, proliferate, and differentiate into various cell types essential for organ development.
Rinkevich et al. (2012) conducted groundbreaking research identifying a mesothelial precursor lineage that gives rise to smooth muscle cells and fibroblasts in mammalian internal organs and their vasculature. This study highlighted the remarkable plasticity of mesothelial cells and their critical contribution to organ development.
Key Factors Involved in MMT:
- Transcription factors (e.g., Snail, Slug, Twist)
- Signaling pathways (e.g., TGF-β, Wnt, Notch)
- Epigenetic regulators
- Extracellular matrix components
The ability of mesothelial cells to undergo MMT is not only crucial for normal development but also has significant implications for pathological processes, including fibrosis and cancer metastasis.
The Role of Mesothelial Cells in Cardiovascular Development
The contribution of mesothelial cells to heart development has been a subject of intense research in recent years. How do these cells participate in the formation of the complex structures of the heart?
Mesothelial cells of the epicardium, the outermost layer of the heart, play a crucial role in cardiac development. Through the process of epithelial-to-mesenchymal transition (EMT), these cells give rise to various cardiac cell types and contribute to the formation of coronary vessels and cardiac valves.
Gittenberger-de Groot et al. (1998) demonstrated that epicardium-derived cells contribute to the myocardial wall and atrioventricular cushions. This pioneering study opened up new avenues for research into the role of mesothelial cells in heart development.
Key Contributions of Epicardial Mesothelial Cells to Heart Development:
- Formation of coronary vasculature
- Development of cardiac fibroblasts
- Contribution to valve formation
- Regulation of myocardial growth and differentiation
Subsequent studies by Cai et al. (2008) and Zhou et al. (2008) provided further evidence for the contribution of epicardial cells to the cardiomyocyte lineage, challenging previous assumptions about the origins of heart muscle cells.
Molecular Mechanisms Governing Mesothelial Cell Function
Understanding the molecular mechanisms that regulate mesothelial cell function is crucial for unraveling their role in development and disease. What are the key genes and signaling pathways involved in mesothelial cell biology?
Several transcription factors and signaling molecules have been identified as crucial regulators of mesothelial cell function. Among these, the Wilms’ tumor 1 (WT1) gene has emerged as a master regulator of mesothelial cell identity and function.
Martínez-Estrada et al. (2010) demonstrated that WT1 is required for cardiovascular progenitor cell formation through transcriptional control of Snail and E-cadherin. This study highlighted the importance of WT1 in regulating the epithelial-to-mesenchymal transition of mesothelial cells.
Key Molecular Players in Mesothelial Cell Biology:
- WT1 (Wilms’ tumor 1)
- Tbx18 (T-box transcription factor 18)
- β-catenin
- Retinoic acid signaling pathway
Research by von Gise et al. (2011) further elucidated the role of WT1 in regulating epicardial epithelial-to-mesenchymal transition through β-catenin and retinoic acid signaling pathways. This study provided insights into the complex molecular networks governing mesothelial cell function.
Mesothelial Cells in Pathological Processes
While mesothelial cells play crucial roles in normal development and tissue homeostasis, they can also contribute to various pathological processes. How do mesothelial cells participate in disease development, and what are the implications for treatment strategies?
Mesothelial cells have been implicated in several pathological conditions, including fibrosis, adhesion formation, and cancer. Their ability to undergo mesothelial-to-mesenchymal transition (MMT) makes them key players in these processes.
Mesothelial Cells in Fibrosis:
In response to injury or inflammation, mesothelial cells can undergo MMT and contribute to the development of fibrosis in various organs. This process involves the production of excessive extracellular matrix components and the release of pro-fibrotic factors.
Mesothelial Cells in Cancer:
Mesothelial cells can be the origin of certain cancers, such as mesothelioma. Additionally, they may contribute to the metastatic spread of other cancers by facilitating the adhesion and invasion of cancer cells into the peritoneal or pleural cavities.
Therapeutic Implications:
Understanding the role of mesothelial cells in pathological processes opens up new avenues for therapeutic interventions. Targeting the molecular pathways involved in MMT or the factors produced by activated mesothelial cells could potentially lead to novel treatments for fibrosis and cancer.
Research into the pathological roles of mesothelial cells is ongoing, with new insights emerging regularly. These findings not only enhance our understanding of disease processes but also pave the way for innovative therapeutic approaches.
Mesothelial to mesenchyme transition as a major developmental and pathological player in trunk organs and their cavities
Wang, N. S. The regional difference of pleural mesothelial cells in rabbits. Am. Rev. Respir. Dis. 110, 623–633 (1974).
CAS
PubMed
Google Scholar
Ishihara, T. et al. Histologic and ultrastructural features of normal human parietal pericardium. Am. J. Cardiol. 46, 744–753 (1980).
CAS
PubMed
Article
Google Scholar
Albertine, K. H., Wiener-Kronish, J. P., Roos, P. J. & Staub, N. C. Structure, blood supply, and lymphatic vessels of the sheep’s visceral pleura. Am. J. Anat. 165, 277–294 (1982).
CAS
PubMed
Article
Google Scholar
Mutsaers, S. E. Mesothelial cells: their structure, function and role in serosal repair. Respirology. 7, 171–191 (2002).
PubMed
Article
Google Scholar
Moore, K., Persaud, T. & Torchia, M. Before We Are Born. Essentials of Embryology and Birth Defects (Elsevier, Oxford, 2015).
Chapter
Google Scholar
Rinkevich, Y. et al. Identification and prospective isolation of a mesothelial precursor lineage giving rise to smooth muscle cells and fibroblasts for mammalian internal organs, and their vasculature. Nat. Cell Biol. 14, 1251–1260 (2012).
CAS
PubMed
PubMed Central
Article
Google Scholar
Gittenberger-de Groot, A. C., Vrancken Peeters, M. P., Mentink, M. M., Gourdie, R. G. & Poelmann, R. E. Epicardium-derived cells contribute a novel population to the myocardial wall and the atrioventricular cushions. Circ. Res. 82, 1043–1052 (1998).
CAS
PubMed
Article
Google Scholar
Pérez-Pomares, J. M. et al. Experimental studies on the spatiotemporal expression of WT1 and RALDh3 in the embryonic avian heart: a model for the regulation of myocardial and valvuloseptal development by epicardially derived cells (EPDCs). Dev. Biol. 247, 307–326 (2002).
PubMed
Article
CAS
Google Scholar
Wilm, B., Ipenberg, A., Hastie, N. D., Burch, J. B. E. & Bader, D. M. The serosal mesothelium is a major source of smooth muscle cells of the gut vasculature. Development 132, 5317–5328 (2005).
CAS
PubMed
Article
Google Scholar
Cai, C.-L. et al. A myocardial lineage derives from Tbx18 epicardial cells. Nature 454, 104–108 (2008).
CAS
PubMed
PubMed Central
Article
Google Scholar
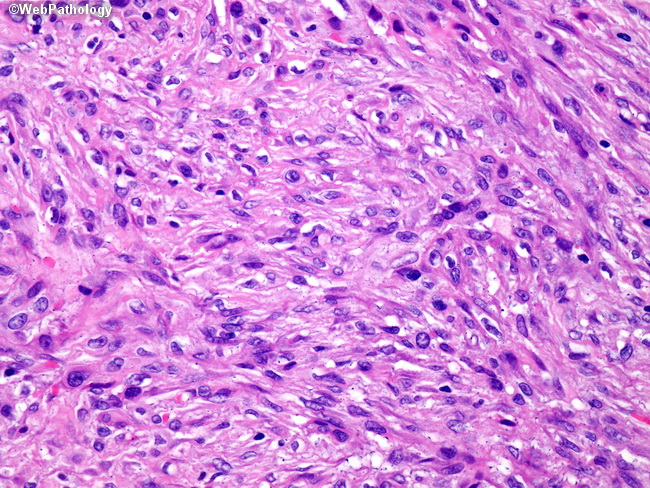
Zhou, B. et al. Epicardial progenitors contribute to the cardiomyocyte lineage in the developing heart. Nature 454, 109–113 (2008).
CAS
PubMed
PubMed Central
Article
Google Scholar
Martínez-Estrada, O. M. et al. Wt1 is required for cardiovascular progenitor cell formation through transcriptional control of Snail and E-cadherin. Nat. Genet. 42, 89–93 (2010).
PubMed
Article
CAS
Google Scholar
Wessels, A. et al. Epicardially derived fibroblasts preferentially contribute to the parietal leaflets of the atrioventricular valves in the murine heart. Dev. Biol. 366, 111–124 (2012).
CAS
PubMed
PubMed Central
Article
Google Scholar
Takeichi, M., Nimura, K., Mori, M., Nakagami, H. & Kaneda, Y. The transcription factors Tbx18 and Wt1 control the epicardial epithelial-mesenchymal transition through bi-directional regulation of Slug in murine primary epicardial cells. PLoS ONE 8, e57829 (2013).
CAS
PubMed
PubMed Central
Article
Google Scholar
Kreidberg, J. A. et al. WT-1 is required for early kidney development. Cell 74, 679–691 (1993).
CAS
Article
Google Scholar
von Gise, A. et al. WT1 regulates epicardial epithelial to mesenchymal transition through β-catenin and retinoic acid signaling pathways. Dev. Biol. 356, 421–431 (2011).
Article
CAS
Google Scholar
Zhou, B., von Gise, A., Ma, Q., Hu, Y. W. & Pu, W. T. Genetic fate mapping demonstrates contribution of epicardium-derived cells to the annulus fibrosis of the mammalian heart. Dev. Biol. 338, 251–261 (2010).
CAS
PubMed
Article
Google Scholar

Zamora, M., Männer, J. & Ruiz-Lozano, P. Epicardium-derived progenitor cells require beta-catenin for coronary artery formation. Proc. Natl Acad. Sci. USA 104, 18109–18114 (2007).
CAS
PubMed
Article
Google Scholar
Acharya, A. et al. The bHLH transcription factor Tcf21 is required for lineage-specific EMT of cardiac fibroblast progenitors. Development 139, 2139–2149 (2012).
CAS
PubMed
PubMed Central
Article
Google Scholar
Braitsch, C. M., Combs, M. D., Quaggin, S. E. & Yutzey, K. E. Pod1/Tcf21 is regulated by retinoic acid signaling and inhibits differentiation of epicardium-derived cells into smooth muscle in the developing heart. Dev. Biol. 368, 345–357 (2012).
CAS
PubMed
PubMed Central
Article
Google Scholar
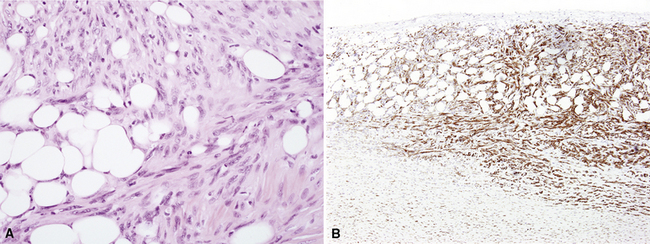
Fraidenraich, D. et al. Rescue of cardiac defects in id knockout embryos by injection of embryonic stem cells. Science 306, 247–252 (2004).
CAS
PubMed
PubMed Central
Article
Google Scholar
Niederreither, K. et al. Embryonic retinoic acid synthesis is essential for heart morphogenesis in the mouse. Development 128, 1019–1031 (2001).
CAS
PubMed
Google Scholar
Guadix, J. A. et al. Wt1 controls retinoic acid signalling in embryonic epicardium through transcriptional activation of Raldh3. Development 138, 1093–1097 (2011).
CAS
PubMed
PubMed Central
Article
Google Scholar
Azambuja, A. P. et al. Retinoic acid and VEGF delay smooth muscle relative to endothelial differentiation to coordinate inner and outer coronary vessel wall morphogenesis. Circ. Res. 107, 204–216 (2010).
CAS
PubMed
Article
Google Scholar
Merki, E. et al. Epicardial retinoid X receptor alpha is required for myocardial growth and coronary artery formation. Proc. Natl Acad. Sci. USA 102, 18455–18460 (2005).
CAS
PubMed
Article
Google Scholar
Watt, A. J., Battle, M. A., Li, J. & Duncan, S. A. GATA4 is essential for formation of the proepicardium and regulates cardiogenesis. Proc. Natl Acad. Sci. USA 101, 12573–12578 (2004).
CAS
PubMed
Article
Google Scholar
Borok, M. J., Papaioannou, V. E. & Sussel, L. Unique functions of Gata4 in mouse liver induction and heart development. Dev. Biol. 410, 213–222 (2016).
CAS
PubMed
Article
Google Scholar
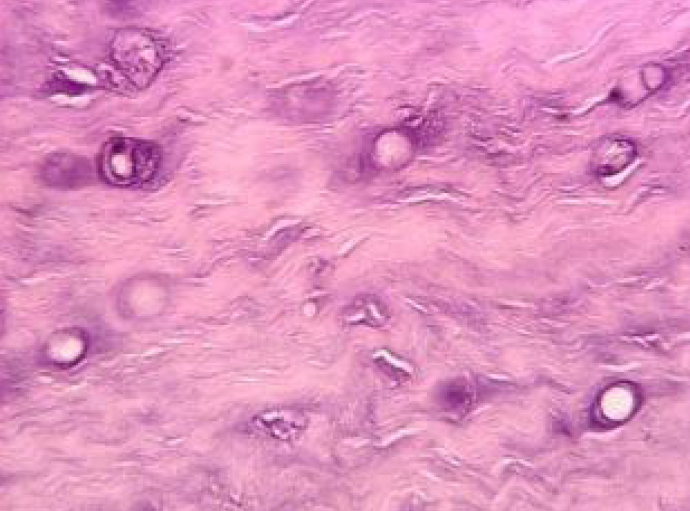
Crispino, J. D. et al. Proper coronary vascular development and heart morphogenesis depend on interaction of GATA-4 with FOG cofactors. Genes Dev. 15, 839–844 (2001).
CAS
PubMed
PubMed Central
Article
Google Scholar
Zhou, B. et al. Fog2 is critical for cardiac function and maintenance of coronary vasculature in the adult mouse heart. J. Clin. Invest. 119, 1462–1476 (2009).
CAS
PubMed
PubMed Central
Article
Google Scholar
Xin, M. et al. A threshold of GATA4 and GATA6 expression is required for cardiovascular development. Proc. Natl Acad. Sci. USA 103, 11189–11194 (2006).
CAS
PubMed
Article
Google Scholar
Kolander, K. D., Holtz, M. L., Cossette, S. M., Duncan, S. A. & Misra, R. P. Epicardial GATA factors regulate early coronary vascular plexus formation. Dev. Biol. 386, 204–215 (2014).
CAS
PubMed
Article
Google Scholar
del Monte, G. et al. Differential Notch signaling in the epicardium is required for cardiac inflow development and coronary vessel morphogenesis. Circ. Res. 108, 824–836 (2011).
PubMed
Article
CAS
Google Scholar
Pérez-Pomares, J. M. et al. Contribution of mesothelium-derived cells to liver sinusoids in avian embryos. Dev. Dyn. 229, 465–474 (2004).
PubMed
Article
Google Scholar
Asahina, K., Zhou, B., Pu, W. T. & Tsukamoto, H. Septum transversum-derived mesothelium gives rise to hepatic stellate cells and perivascular mesenchymal cells in developing mouse liver. Hepatology 53, 983–995 (2011).
CAS
PubMed
PubMed Central
Article
Google Scholar

Lua, I., James, D., Wang, J., Wang, K. S. & Asahina, K. Mesodermal mesenchymal cells give rise to myofibroblasts, but not epithelial cells, in mouse liver injury. Hepatology 60, 311–322 (2014).
CAS
PubMed
PubMed Central
Article
Google Scholar
Delgado, I. et al. GATA4 loss in the septum transversum mesenchyme promotes liver fibrosis in mice. Hepatology 59, 2358–2370 (2014).
CAS
PubMed
Article
Google Scholar
Ijpenberg, A. et al. Wt1 and retinoic acid signaling are essential for stellate cell development and liver morphogenesis. Dev. Biol. 312, 157–170 (2007).
CAS
PubMed
Article
Google Scholar
Paris, N. D., Coles, G. L. & Ackerman, K. G. Wt1 and β-catenin cooperatively regulate diaphragm development in the mouse. Dev. Biol. 407, 40–56 (2015).
CAS
PubMed
PubMed Central
Article
Google Scholar
Berg, T. et al. β-catenin regulates mesenchymal progenitor cell differentiation during hepatogenesis. J. Surg. Res. 164, 276–285 (2010).
CAS
PubMed
Article
Google Scholar
Watt, A. J., Zhao, R., Li, J. & Duncan, S. A. Development of the mammalian liver and ventral pancreas is dependent on GATA4. BMC Dev. Biol. 7, 37 (2007).
PubMed
PubMed Central
Article
CAS
Google Scholar
Miyamoto, Y., Taniguchi, H., Hamel, F., Silversides, D. W. & Viger, R. S. A GATA4/WT1 cooperation regulates transcription of genes required for mammalian sex determination and differentiation. BMC Mol. Biol. 9, 44 (2008).
PubMed
PubMed Central
Article
CAS
Google Scholar

Ikeda, Y., Shen, W. H., Ingraham, H. A. & Parker, K. L. Developmental expression of mouse steroidogenic factor-1, an essential regulator of the steroid hydroxylases . Mol. Endocrinol. 8, 654–662 (1994).
CAS
PubMed
Google Scholar
Hatano, O., Takakusu, A., Nomura, M. & Morohashi, K. Identical origin of adrenal cortex and gonad revealed by expression profiles of Ad4BP/SF-1. Genes Cells 1, 663–671 (1996).
CAS
PubMed
Article
Google Scholar
Karl, J. & Capel, B. Sertoli cells of the mouse testis originate from the coelomic epithelium. Dev. Biol. 203, 323–333 (1998).
CAS
PubMed
Article
Google Scholar
Hammes, A. et al. Two splice variants of the Wilms’ tumor 1 gene have distinct functions during sex determination and nephron formation. Cell 106, 319–329 (2001).
CAS
Article
Google Scholar
Liu, C., Peng, J., Matzuk, M. M. & Yao, H. H.-C. Lineage specification of ovarian theca cells requires multicellular interactions via oocyte and granulosa cells. Nat. Commun. 6, 6934 (2015).
CAS
PubMed
PubMed Central
Article
Google Scholar
Bohnenpoll, T. et al. Tbx18 expression demarcates multipotent precursor populations in the developing urogenital system but is exclusively required within the ureteric mesenchymal lineage to suppress a renal stromal fate. Dev. Biol. 380, 25–36 (2013).
CAS
PubMed
Article
Google Scholar
Häfner, R., Bohnenpoll, T., Rudat, C., Schultheiss, T. M. & Kispert, A. Fgfr2 is required for the expansion of the early adrenocortical primordium. Mol. Cell Endocrinol. 413, 168–177 (2015).
PubMed
Article
CAS
Google Scholar
Bingham, N. C., Verma-Kurvari, S., Parada, L. F. & Parker, K. L. Development of a steroidogenic factor 1/Cre transgenic mouse line. Genesis 44, 419–424 (2006).
CAS
PubMed
Article
Google Scholar
Kusaka, M. et al. Abnormal epithelial cell polarity and ectopic epidermal growth factor receptor (EGFR) expression induced in Emx2 KO embryonic gonads. Endocrinology 151, 5893–5904 (2010).
CAS
PubMed
Article
Google Scholar
Hummitzsch, K. et al. A new model of development of the mammalian ovary and follicles. PLoS ONE 8, e55578 (2013).
CAS
PubMed
PubMed Central
Article
Google Scholar

Albrecht, K. H. & Eicher, E. M. Evidence that Sry is expressed in pre-Sertoli cells and Sertoli and granulosa cells have a common precursor. Dev. Biol. 240, 92–107 (2001).
CAS
PubMed
Article
Google Scholar
Combes, A. N. et al. Endothelial cell migration directs testis cord formation. Dev. Biol. 326, 112–120 (2009).
CAS
PubMed
Article
Google Scholar
Chassot, A.-A. et al. Activation of beta-catenin signaling by Rspo1 controls differentiation of the mammalian ovary. Hum. Mol. Genet. 17, 1264–1277 (2008).
CAS
PubMed
Article
Google Scholar
Piprek, R. P. Molecular mechanisms underlying female sex determination—antagonism between female and male pathway. Folia Biol. (Praha) 57, 105–113 (2009).
CAS
Article
Google Scholar
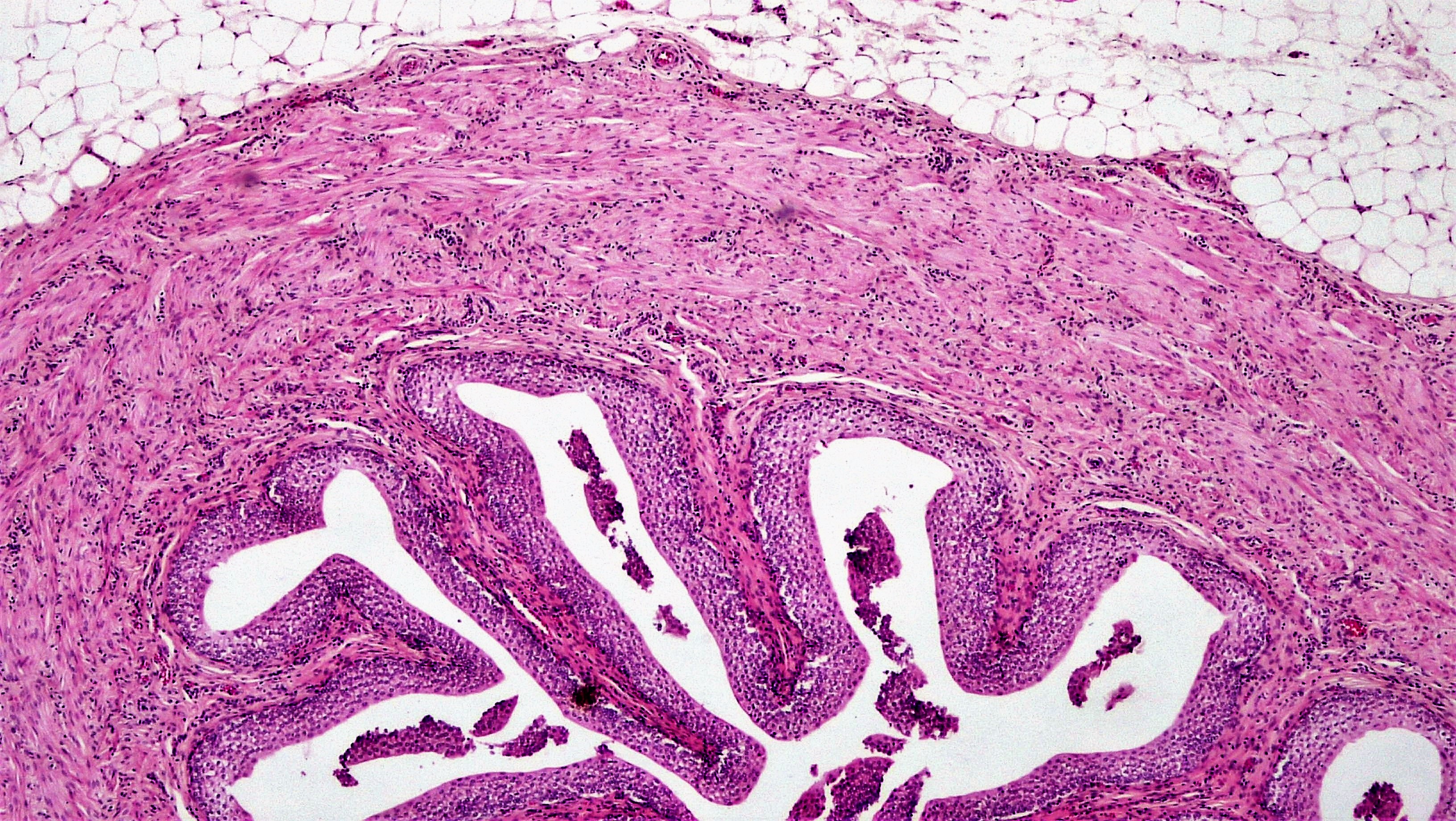
Brennan, J., Tilmann, C. & Capel, B. Pdgfr-alpha mediates testis cord organization and fetal Leydig cell development in the XY gonad. Genes Dev. 17, 800–810 (2003).
CAS
PubMed
PubMed Central
Article
Google Scholar
Cool, J., Carmona, F. D., Szucsik, J. C. & Capel, B. Peritubular myoid cells are not the migrating population required for testis cord formation in the XY gonad. Sex. Dev. 2, 128–133 (2008).
CAS
PubMed
PubMed Central
Article
Google Scholar
Barsoum, I. B., Kaur, J., Ge, R. S., Cooke, P. S. & Yao, H. H.-C. Dynamic changes in fetal Leydig cell populations influence adult Leydig cell populations in mice.FASEB J. 27, 2657–2666 (2013).
CAS
PubMed
PubMed Central
Article
Google Scholar
Hu, Y. -C., Okumura, L. M. & Page, D. C. Gata4 is required for formation of the genital ridge in mice. PLoS Genet. 9, e1003629 (2013).
CAS
PubMed
PubMed Central
Article
Google Scholar
Airik, R., Bussen, M., Singh, M. K., Petry, M. & Kispert, A. Tbx18 regulates the development of the ureteral mesenchyme. J. Clin. Invest. 116, 663–674 (2006).
CAS
PubMed
PubMed Central
Article
Google Scholar
Cui, S. et al. Disrupted gonadogenesis and male-to-female sex reversal in Pod1 knockout mice. Development 131, 4095–4105 (2004).
CAS
PubMed
Article
Google Scholar
Tevosian, S. G. et al. Gonadal differentiation, sex determination and normal Sry expression in mice require direct interaction between transcription partners GATA4 and FOG2. Development 129, 4627–4634 (2002).
CAS
PubMed
Google Scholar
Wilhelm, D. & Englert, C. The Wilms tumor suppressor WT1 regulates early gonad development by activation of Sf1. Genes Dev. 16, 1839–1851 (2002).
CAS
PubMed
PubMed Central
Article
Google Scholar
Fujimoto, Y. et al. Homeoproteins Six1 and Six4 regulate male sex determination and mouse gonadal development. Dev. Cell 26, 416–430 (2013).
CAS
PubMed
Article
Google Scholar
Klattig, J., Sierig, R., Kruspe, D., Makki, M. S. & Englert, C. WT1-mediated gene regulation in early urogenital ridge development. Sex. Dev. 1, 238–254 (2007).
CAS
PubMed
Article
Google Scholar
Ponnusamy, M. P. et al. Emerging role of mucins in epithelial to mesenchymal transition. Curr. Cancer Drug Targets 13, 945–956 (2013).
CAS
PubMed
PubMed Central
Article
Google Scholar
Chen, S.-H., Hung, W.-C., Wang, P., Paul, C. & Konstantopoulos, K. Mesothelin binding to CA125/MUC16 promotes pancreatic cancer cell motility and invasion via MMP-7 activation. Sci. Rep. 3, 1870 (2013).
PubMed
PubMed Central
Article
CAS
Google Scholar
Lécureuil, C., Fontaine, I., Crepieux, P. & Guillou, F. Sertoli and granulosa cell-specific Cre recombinase activity in transgenic mice. Genesis 33, 114–118 (2002).
PubMed
Article
CAS
Google Scholar
Gao, F. et al. The Wilms tumor gene, Wt1, is required for Sox9 expression and maintenance of tubular architecture in the developing testis. Proc. Natl Acad. Sci. USA 103, 11987–11992 (2006).
CAS
PubMed
Article
Google Scholar
Wen, Q., Wang, Y., Tang, J., Cheng, C. Y. & Liu, Y.-X. Sertoli cell Wt1 regulates peritubular myoid cell and fetal Leydig cell differentiation during fetal testis development. PLoS ONE 11, e0167920 (2016).
PubMed
PubMed Central
Article
CAS
Google Scholar
Chen, M. et al. Wt1 directs the lineage specification of sertoli and granulosa cells by repressing Sf1 expression. Development 144, 44–53 (2017).
CAS
PubMed
Article
Google Scholar
Zhang, L. et al. Reprogramming of Sertoli cells to fetal-like Leydig cells by Wt1 ablation. Proc. Natl Acad. Sci. USA 112, 4003–4008 (2015).
CAS
PubMed
Article
Google Scholar

Bandiera, R. et al. WT1 maintains adrenal-gonadal primordium identity and marks a population of AGP-like progenitors within the adrenal gland. Dev. Cell 27, 5–18 (2013).
CAS
PubMed
PubMed Central
Article
Google Scholar
Hogan, B. L. Morphogenesis. Cell 96, 225–233 (1999).
CAS
PubMed
Article
Google Scholar
Norden, J., Grieskamp, T., Christoffels, V. M., Moorman, A. F. M. & Kispert, A. Partial absence of pleuropericardial membranes in Tbx18- and Wt1-deficient mice. PLoS ONE 7, e45100 (2012).
CAS
PubMed
PubMed Central
Article
Google Scholar
Malpel, S., Mendelsohn, C. & Cardoso, W. V. Regulation of retinoic acid signaling during lung morphogenesis. Development 127, 3057–3067 (2000).
CAS
PubMed
Google Scholar
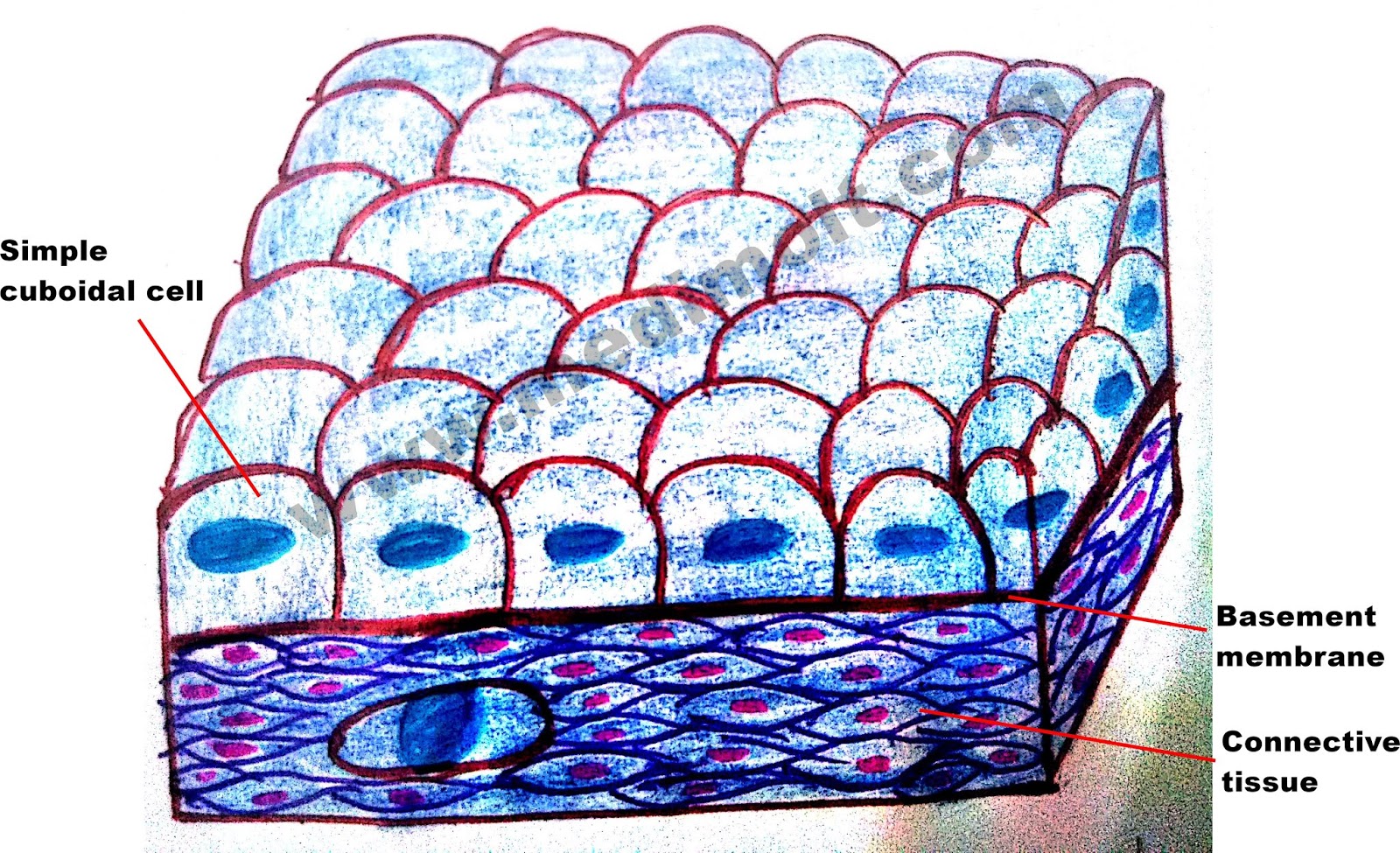
Niederreither, K., Fraulob, V., Garnier, J.-M., Chambon, P. & Dollé, P. Differential expression of retinoic acid-synthesizing (RALDH) enzymes during fetal development and organ differentiation in the mouse. Mech. Dev. 110, 165–171 (2002).
CAS
PubMed
Article
Google Scholar
Cano, E., Carmona, R. & Muñoz-Chápuli, R. Wt1-expressing progenitors contribute to multiple tissues in the developing lung. Am. J. Physiol. Lung Cell Mol. Physiol. 305, L322–L332 (2013).
CAS
PubMed
Article
Google Scholar
Morimoto, M. et al. Canonical Notch signaling in the developing lung is required for determination of arterial smooth muscle cells and selection of Clara versus ciliated cell fate. J. Cell Sci. 123, 213–224 (2010).
CAS
PubMed
PubMed Central
Article
Google Scholar

Kaarteenaho, R., Lappi-Blanco, E. & Lehtonen, S. Epithelial N-cadherin and nuclear β-catenin are up-regulated during early development of human lung. BMC Dev. Biol. 10, 113 (2010).
PubMed
PubMed Central
Article
CAS
Google Scholar
Ackerman, K. G. et al. Gata4 is necessary for normal pulmonary lobar development. Am. J. Respir. Cell Mol. Biol. 36, 391–397 (2007).
CAS
PubMed
Article
Google Scholar
Dixit, R., Ai, X. & Fine, A. Derivation of lung mesenchymal lineages from the fetal mesothelium requires hedgehog signaling for mesothelial cell entry. Development 140, 4398–4406 (2013).
CAS
PubMed
PubMed Central
Article
Google Scholar
Que, J. et al. Mesothelium contributes to vascular smooth muscle and mesenchyme during lung development. Proc. Natl Acad. Sci. USA 105, 16626–16630 (2008).
CAS
PubMed
Article
Google Scholar
von Gise, A. et al. Contribution of fetal, but not adult, pulmonary mesothelium to mesenchymal lineages in lung homeostasis and fibrosis. Am. J. Respir. Cell Mol. Biol. 54, 222–230 (2016).
Article
CAS
Google Scholar
Norden, J. et al. Wt1 and retinoic acid signaling in the subcoelomic mesenchyme control the development of the pleuropericardial membranes and the sinus horns. Circ. Res. 106, 1212–1220 (2010).
CAS
PubMed
PubMed Central
Article
Google Scholar
Ackerman, K. G. et al. Fog2 is required for normal diaphragm and lung development in mice and humans. PLoS Genet. 1, 58–65 (2005).
CAS
PubMed
Article
Google Scholar

Jay, P. Y. et al. Impaired mesenchymal cell function in Gata4 mutant mice leads to diaphragmatic hernias and primary lung defects. Dev. Biol. 301, 602–614 (2007).
CAS
PubMed
Article
Google Scholar
Shikama, N. et al. Essential function of p300 acetyltransferase activity in heart, lung and small intestine formation. EMBO J. 22, 5175–5185 (2003).
CAS
PubMed
PubMed Central
Article
Google Scholar
Yin, Y. et al. An FGF-WNT gene regulatory network controls lung mesenchyme development. Dev. Biol. 319, 426–436 (2008).
CAS
PubMed
PubMed Central
Article
Google Scholar
De Langhe, S. P. et al. Formation and differentiation of multiple mesenchymal lineages during lung development is regulated by beta-catenin signaling. PLoS ONE 3, e1516 (2008).
PubMed
PubMed Central
Article
CAS
Google Scholar
Desai, T. J. et al. Distinct roles for retinoic acid receptors alpha and beta in early lung morphogenesis. Dev. Biol. 291, 12–24 (2006).
CAS
PubMed
Article
Google Scholar
Wang, Z., Dollé, P., Cardoso, W. V. & Niederreither, K. Retinoic acid regulates morphogenesis and patterning of posterior foregut derivatives. Dev. Biol. 297, 433–445 (2006).
CAS
PubMed
Article
Google Scholar
Chazaud, C., Dollé, P., Rossant, J. & Mollard, R. Retinoic acid signaling regulates murine bronchial tubule formation. Mech. Dev. 120, 691–700 (2003).
CAS
PubMed
Article
Google Scholar
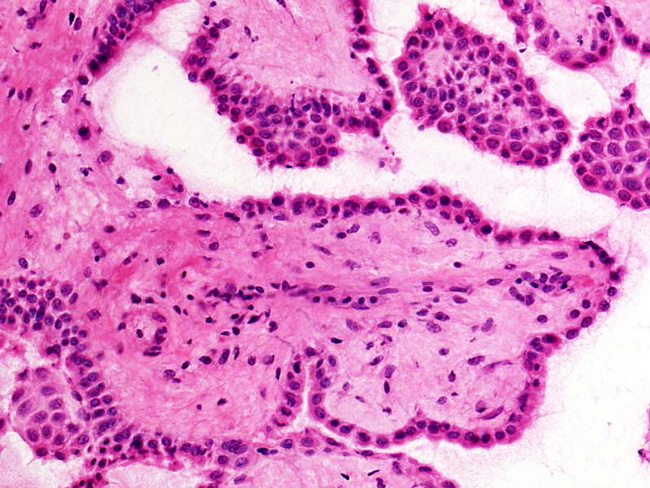
Cardenas, H., Zhao, J., Vieth, E., Nephew, K. P. & Matei, D. EZh3 inhibition promotes epithelial-to-mesenchymal transition in ovarian cancer cells. Oncotarget 7, 84453–84467 (2016).
PubMed
PubMed Central
Article
Google Scholar
Snitow, M., Lu, M., Cheng, L., Zhou, S. & Morrisey, E. E. Ezh3 restricts the smooth muscle lineage during mouse lung mesothelial development. Development 143, 3733–3741 (2016).
CAS
PubMed
PubMed Central
Article
Google Scholar
Zhou, B. et al. Adult mouse epicardium modulates myocardial injury by secreting paracrine factors. J. Clin. Invest. 121, 1894–1904 (2011).
CAS
PubMed
PubMed Central
Article
Google Scholar
Huang, G. N. et al. C/EBP transcription factors mediate epicardial activation during heart development and injury. Science 338, 1599–1603 (2012).
CAS
PubMed
PubMed Central
Article
Google Scholar
Limana, F. et al. Identification of myocardial and vascular precursor cells in human and mouse epicardium. Circ. Res. 101, 1255–1265 (2007).
CAS
PubMed
Article
Google Scholar
Limana, F. et al. Myocardial infarction induces embryonic reprogramming of epicardial c-kit(+) cells: role of the pericardial fluid. J. Mol. Cell Cardiol. 48, 609–618 (2010).
CAS
PubMed
Article
Google Scholar
Kikuchi, K. et al. Retinoic acid production by endocardium and epicardium is an injury response essential for zebrafish heart regeneration. Dev. Cell 20, 397–404 (2011).
CAS
PubMed
PubMed Central
Article
Google Scholar
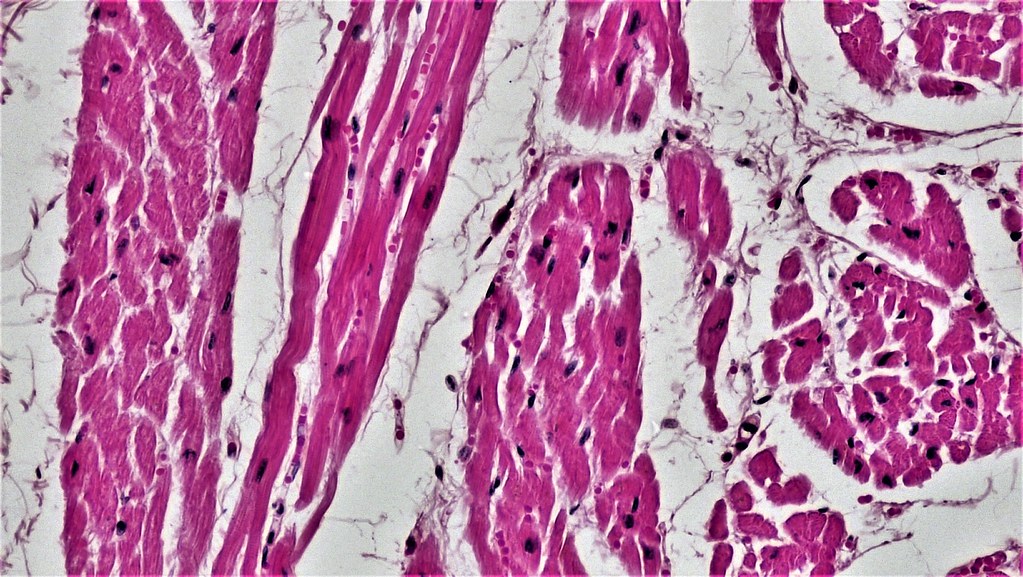
Duan, J. et al. Wnt1/βcatenin injury response activates the epicardium and cardiac fibroblasts to promote cardiac repair. EMBO J. 31, 429–442 (2012).
CAS
PubMed
Article
Google Scholar
Ruiz-Villalba, A. et al. Interacting resident epicardium-derived fibroblasts and recruited bone marrow cells form myocardial infarction scar. J. Am. Coll. Cardiol. 65, 2057–2066 (2015).
PubMed
Article
Google Scholar
Wang, J., Cao, J., Dickson, A. L. & Poss, K. D. Epicardial regeneration is guided by cardiac outflow tract and Hedgehog signalling. Nature 522, 226–230 (2015).
CAS
PubMed
PubMed Central
Article
Google Scholar
Cao, J. et al. Single epicardial cell transcriptome sequencing identifies Caveolin 1 as an essential factor in zebrafish heart regeneration. Development 143, 232–243 (2016).
CAS
PubMed
PubMed Central
Article
Google Scholar
Bock-Marquette, I. et al. Thymosin beta4 mediated PKC activation is essential to initiate the embryonic coronary developmental program and epicardial progenitor cell activation in adult mice in vivo. J. Mol. Cell Cardiol. 46, 728–738 (2009).
CAS
PubMed
PubMed Central
Article
Google Scholar
Smart, N. et al. Thymosin beta4 facilitates epicardial neovascularization of the injured adult heart. Ann. NY Acad. Sci. 1194, 97–104 (2010).
CAS
PubMed
Article
Google Scholar
Moore-Morris, T. et al. Resident fibroblast lineages mediate pressure overload-induced cardiac fibrosis. J. Clin. Invest. 124, 2921–2934 (2014).
CAS
PubMed
PubMed Central
Article
Google Scholar
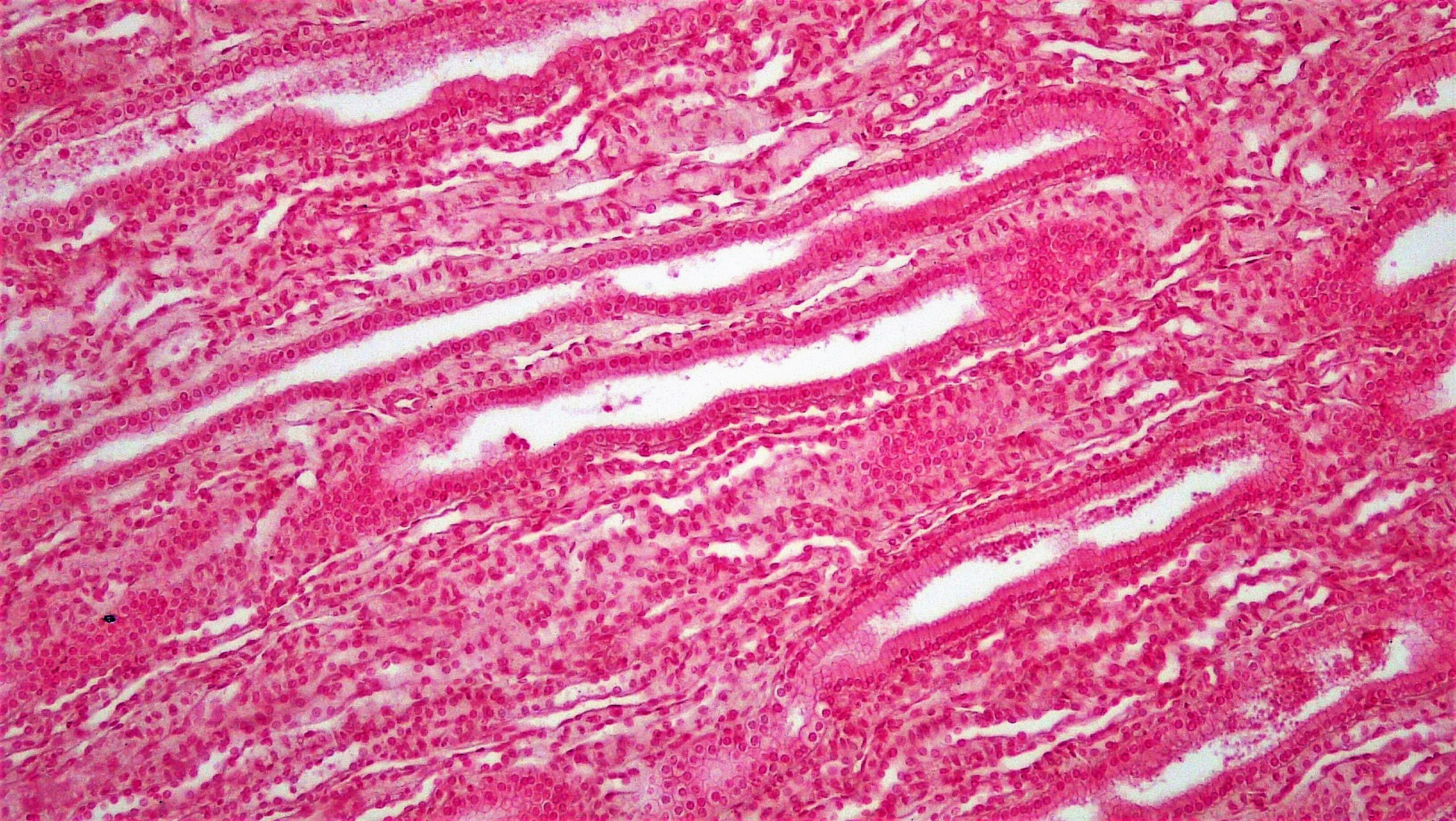
Ali, S. R. et al. Developmental heterogeneity of cardiac fibroblasts does not predict pathological proliferation and activation. Circ. Res. 115, 625–635 (2014).
CAS
PubMed
Article
Google Scholar
Braitsch, C. M., Kanisicak, O., van Berlo, J. H., Molkentin, J. D. & Yutzey, K. E. Differential expression of embryonic epicardial progenitor markers and localization of cardiac fibrosis in adult ischemic injury and hypertensive heart disease. J. Mol. Cell Cardiol. 65, 108–119 (2013).
CAS
PubMed
Article
Google Scholar
Li, Y., Wang, J. & Asahina, K. Mesothelial cells give rise to hepatic stellate cells and myofibroblasts via mesothelial-mesenchymal transition in liver injury. Proc. Natl Acad. Sci. USA 110, 2324–2329 (2013).
CAS
PubMed
Article
Google Scholar
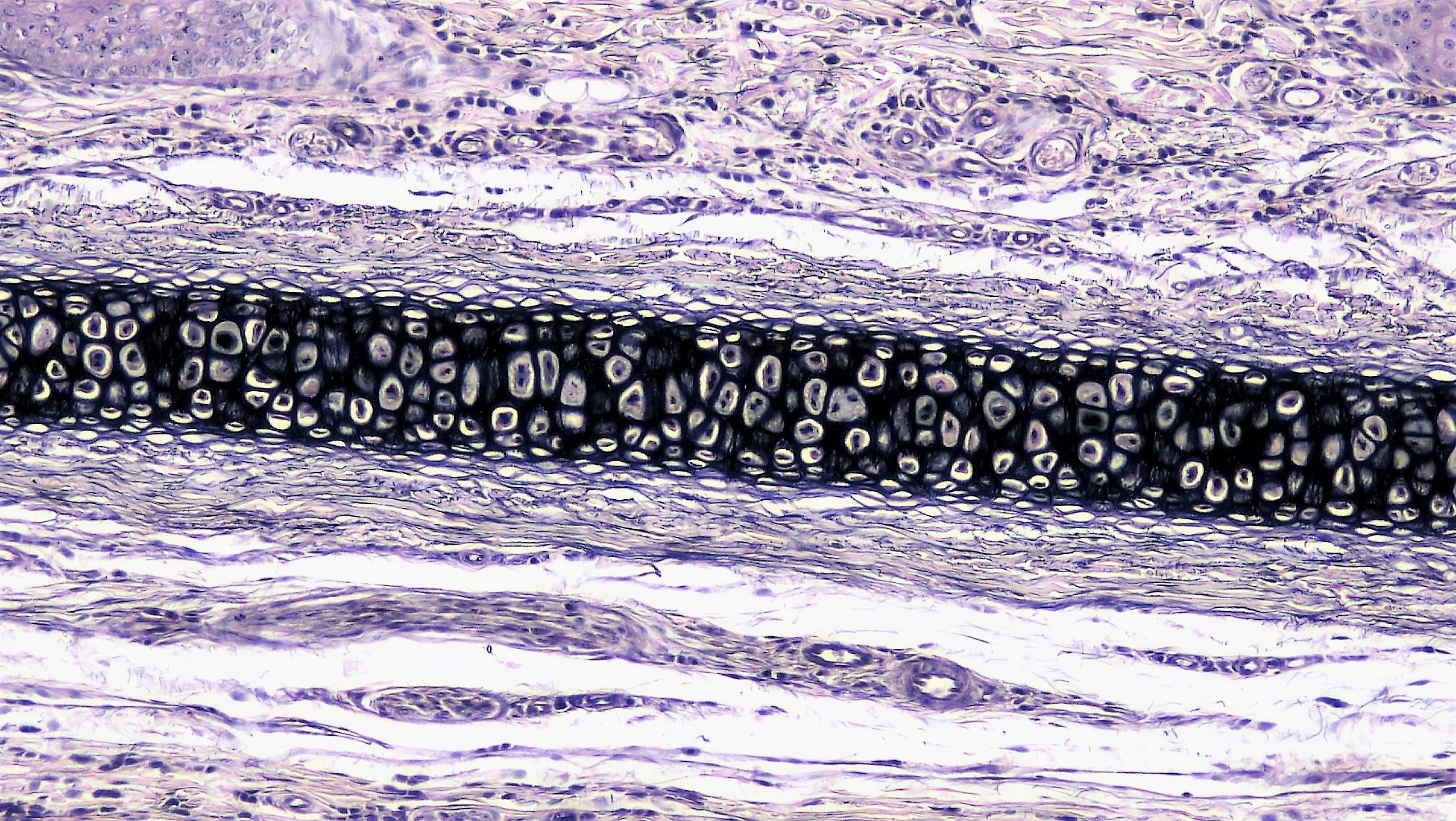
Asahina, K. et al. Mesenchymal origin of hepatic stellate cells, submesothelial cells, and perivascular mesenchymal cells during mouse liver development. Hepatology 49, 998–1011 (2009).
CAS
PubMed
PubMed Central
Article
Google Scholar
Iwaisako, K. et al. Origin of myofibroblasts in the fibrotic liver in mice. Proc. Natl Acad. Sci. USA 111, E3297–E3305 (2014).
CAS
PubMed
Article
Google Scholar
Koyama, Y. et al. Mesothelin/mucin 16 signaling in activated portal fibroblasts regulates cholestatic liver fibrosis. J. Clin. Invest. 127, 1254–1270 (2017).
PubMed
PubMed Central
Article
Google Scholar
Ochiai, K., Muramatsu, H., Yamamoto, S., Ando, H. & Muramatsu, T. The role of midkine and pleiotrophin in liver regeneration. Liver Int. 24, 484–491 (2004).
CAS
PubMed
Article
Google Scholar
Inagaki, N. F., Inagaki, F. F., Kokudo, N. & Miyajima, A. Use of mouse liver mesothelial cells to prevent postoperative adhesion and promote liver regeneration after hepatectomy. J. Hepatol. 62, 1141–1147 (2015).
CAS
PubMed
Article
Google Scholar
Miyoshi, H., Ajima, R., Luo, C. T., Yamaguchi, T. P. & Stappenbeck, T. S. Wnt5a potentiates TGF-β signaling to promote colonic crypt regeneration after tissue injury. Science 338, 108–113 (2012).
CAS
PubMed
PubMed Central
Article
Google Scholar
Smart, N. et al. Thymosin beta4 induces adult epicardial progenitor mobilization and neovascularization. Nature 445, 177–182 (2007).
CAS
PubMed
Article
Google Scholar
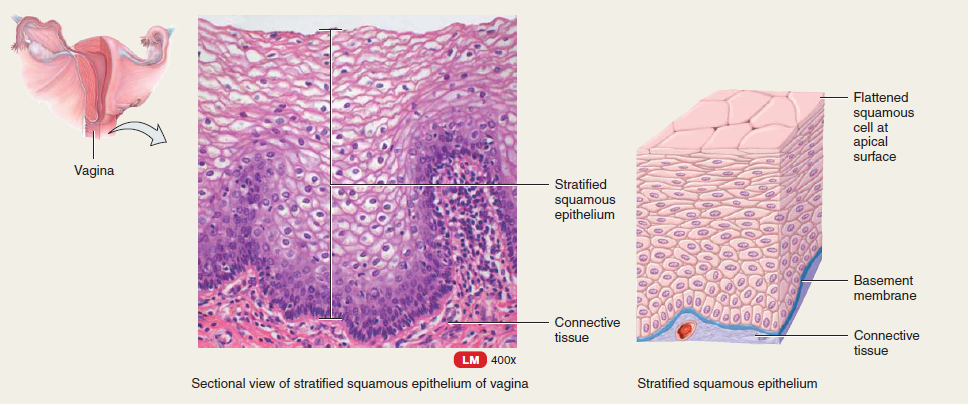
Auersperg, N., Wong, A. S., Choi, K. C., Kang, S. K. & Leung, P. C. Ovarian surface epithelium: biology, endocrinology, and pathology. Endocr. Rev. 22, 255–288 (2001).
CAS
PubMed
Google Scholar
Ahmed, N. et al. Molecular pathways regulating EGF-induced epithelio-mesenchymal transition in human ovarian surface epithelium. Am. J. Physiol. Cell Physiol. 290, C1532–C1542 (2006).
CAS
PubMed
Article
Google Scholar
Zhu, Y., Nilsson, M. & Sundfeldt, K. Phenotypic plasticity of the ovarian surface epithelium: TGF-beta 1 induction of epithelial to mesenchymal transition (EMT) in vitro. Endocrinology 151, 5497–5505 (2010).
CAS
PubMed
Article
Google Scholar
Flesken-Nikitin, A. et al. Ovarian surface epithelium at the junction area contains a cancer-prone stem cell niche. Nature 495, 241–245 (2013).
CAS
PubMed
PubMed Central
Article
Google Scholar
Gamwell, L. F., Collins, O. & Vanderhyden, B. C. The mouse ovarian surface epithelium contains a population of LY6A (SCA-1) expressing progenitor cells that are regulated by ovulation-associated factors. Biol. Reprod. 87, 80 (2012).
PubMed
Article
Google Scholar
Szotek, P. P. et al. Normal ovarian surface epithelial label-retaining cells exhibit stem/progenitor cell characteristics. Proc. Natl Acad. Sci. USA 105, 12469–12473 (2008).
CAS
PubMed
Article
Google Scholar
Beller, U., Haimovitch, R. & Ben-Sasson, S. Periovulatory multifocal mesothelial proliferation: a possible association with malignant transformation. Int. J. Gynecol. Cancer 5, 306–309 (1995).
PubMed
Article
Google Scholar
Tan, O. L. & Fleming, J. S. Proliferating cell nuclear antigen immunoreactivity in the ovarian surface epithelium of mice of varying ages and total lifetime ovulation number following ovulation. Biol. Reprod. 71, 1501–1507 (2004).
CAS
PubMed
Article
Google Scholar
Ng, A. et al. Lgr5 marks stem/progenitor cells in ovary and tubal epithelia. Nat. Cell Biol. 16, 745–757 (2014).
CAS
PubMed
Article
Google Scholar
Batra, H. & Antony, V. B. Pleural mesothelial cells in pleural and lung diseases. J. Thorac. Dis. 7, 964–980 (2015).
PubMed
PubMed Central
Google Scholar
Huggins, J. T. & Sahn, S. A. Causes and management of pleural fibrosis. Respirology 9, 441–447 (2004).
PubMed
Article
Google Scholar
Chen, L.-J. et al. Bleomycin induced epithelial-mesenchymal transition (EMT) in pleural mesothelial cells. Toxicol. Appl. Pharmacol. 283, 75–82 (2015).
CAS
PubMed
Article
Google Scholar
Zolak, J. S. et al. Pleural mesothelial cell differentiation and invasion in fibrogenic lung injury. Am. J. Pathol. 182, 1239–1247 (2013).
CAS
PubMed
PubMed Central
Article
Google Scholar
Zhang, Q. et al. miR-18a-5p inhibits sub-pleural pulmonary fibrosis by targeting TGF-β receptor II. Mol. Ther. 25, 728–738 (2017).
CAS
PubMed
PubMed Central
Article
Google Scholar

Decologne, N. et al. Bleomycin induces pleural and subpleural fibrosis in the presence of carbon particles. Eur. Respir. J. 35, 176–185 (2010).
CAS
PubMed
Article
Google Scholar
Tucker, T. A. et al. Plasminogen activator inhibitor-1 deficiency augments visceral mesothelial organization, intrapleural coagulation, and lung restriction in mice with carbon black/bleomycin-induced pleural injury. Am. J. Respir. Cell Mol. Biol. 50, 316–327 (2014).
PubMed
PubMed Central
Google Scholar
Boren, J. et al. Inhibition of glycogen synthase kinase 3β blocks mesomesenchymal transition and attenuates Streptococcus pneumonia-mediated pleural injury in mice. Am. J. Pathol. 187, 2461–2472 (2017).
CAS
PubMed
Article
Google Scholar
Cool, C. D. et al. Fibroblast foci are not discrete sites of lung injury or repair: the fibroblast reticulum. Am. J. Respir. Crit. Care Med. 174, 654–658 (2006).
PubMed
PubMed Central
Article
Google Scholar
Wellman, T. J., Mondoñedo, J. R., Davis, G. S., Bates, J. H. T. & Suki, B. Topographic distribution of idiopathic pulmonary fibrosis: a hybrid physics- and agent-based model. Physiol. Meas. 39, 064007 (2018).
PubMed
Article
Google Scholar
Mubarak, K. K. et al. Parenchymal trafficking of pleural mesothelial cells in idiopathic pulmonary fibrosis. Eur. Respir. J. 39, 133–140 (2012).
CAS
PubMed
Article
Google Scholar
Karki, S. Wilms’ tumor 1 (Wt1) regulates pleural mesothelial cell plasticity and transition into myofibroblasts in idiopathic pulmonary fibrosis. FASEB J. 28, 1122–1131 (2014).
CAS
PubMed
PubMed Central
Article
Google Scholar
Decologne, N. et al. TGF-beta1 induces progressive pleural scarring and subpleural fibrosis. J. Immunol. 179, 6043–6051 (2007).
CAS
PubMed
Article
Google Scholar
Suzuki, T. et al. An injured tissue affects the opposite intact peritoneum during postoperative adhesion formation. Sci. Rep. 5, 7668 (2015).
CAS
PubMed
PubMed Central
Article
Google Scholar
Stangel, J. J., Nisbet, J. D. & Settles, H. Formation and prevention of postoperative abdominal adhesions. J. Reprod. Med. 29, 143–156 (1984).
CAS
PubMed
Google Scholar
Rocca, A. et al. Prevention and treatment of peritoneal adhesions in patients affected by vascular diseases following surgery: a review of the literature. Open Med. Wars. Pol. 11, 106–114 (2016).
CAS
Google Scholar
Liu, Y. et al. Transition of mesothelial cell to fibroblast in peritoneal dialysis: EMT, stem cell or bystander? Perit. Dial. Int. 35, 14–25 (2015).
CAS
PubMed
PubMed Central
Article
Google Scholar
Di Paolo, N. & Sacchi, G. Peritoneal vascular changes in continuous ambulatory peritoneal dialysis (CAPD): an in vivo model for the study of diabetic microangiopathy. Perit. Dial. Int. 9, 41–45 (1989).
PubMed
Google Scholar
Honda, K., Nitta, K., Horita, S., Yumura, W. & Nihei, H. Morphological changes in the peritoneal vasculature of patients on CAPD with ultrafiltration failure. Nephron 72, 171–176 (1996).
CAS
PubMed
Article
Google Scholar

Di Paolo, N. et al. Morphology of the peritoneal membrane during continuous ambulatory peritoneal dialysis. Nephron 44, 204–211 (1986).
PubMed
Article
Google Scholar
Mateijsen, M. A. et al. Vascular and interstitial changes in the peritoneum of CAPD patients with peritoneal sclerosis. Perit. Dial. Int. 19, 517–525 (1999).
CAS
PubMed
Google Scholar
Kawaguchi, Y., Kawanishi, H., Mujais, S., Topley, N. & Oreopoulos, D. G. Encapsulating peritoneal sclerosis: definition, etiology, diagnosis, and treatment. International Society for Peritoneal Dialysis Ad Hoc Committee on Ultrafiltration Management in Peritoneal Dialysis. Perit. Dial. Int. 20(Suppl 4), S43–S55 (2000).
PubMed
Google Scholar
Williams, J. D. et al. The natural course of peritoneal membrane biology during peritoneal dialysis. Kidney Int. Suppl. 64, S43–S49 (2003).
Whitaker, D., Manning, L., Robinson, B. & Shilkin, K. in The Pathobiology of the Mesothelium 25–68 (Hemisphere Publishing Corp., New York, 1992).
Yang, A. H., Chen, J. Y. & Lin, J. K. Myofibroblastic conversion of mesothelial cells. Kidney Int. 63, 1530–1539 (2003).
PubMed
Article
Google Scholar
Yáñez-Mó, M. et al. Peritoneal dialysis and epithelial-to-mesenchymal transition of mesothelial cells. N. Engl. J. Med. 348, 403–413 (2003).
PubMed
Article
Google Scholar
Sandoval, P. et al. Mesothelial-to-mesenchymal transition in the pathogenesis of post-surgical peritoneal adhesions. J. Pathol. 239, 48–59 (2016).
CAS
PubMed
Article
Google Scholar
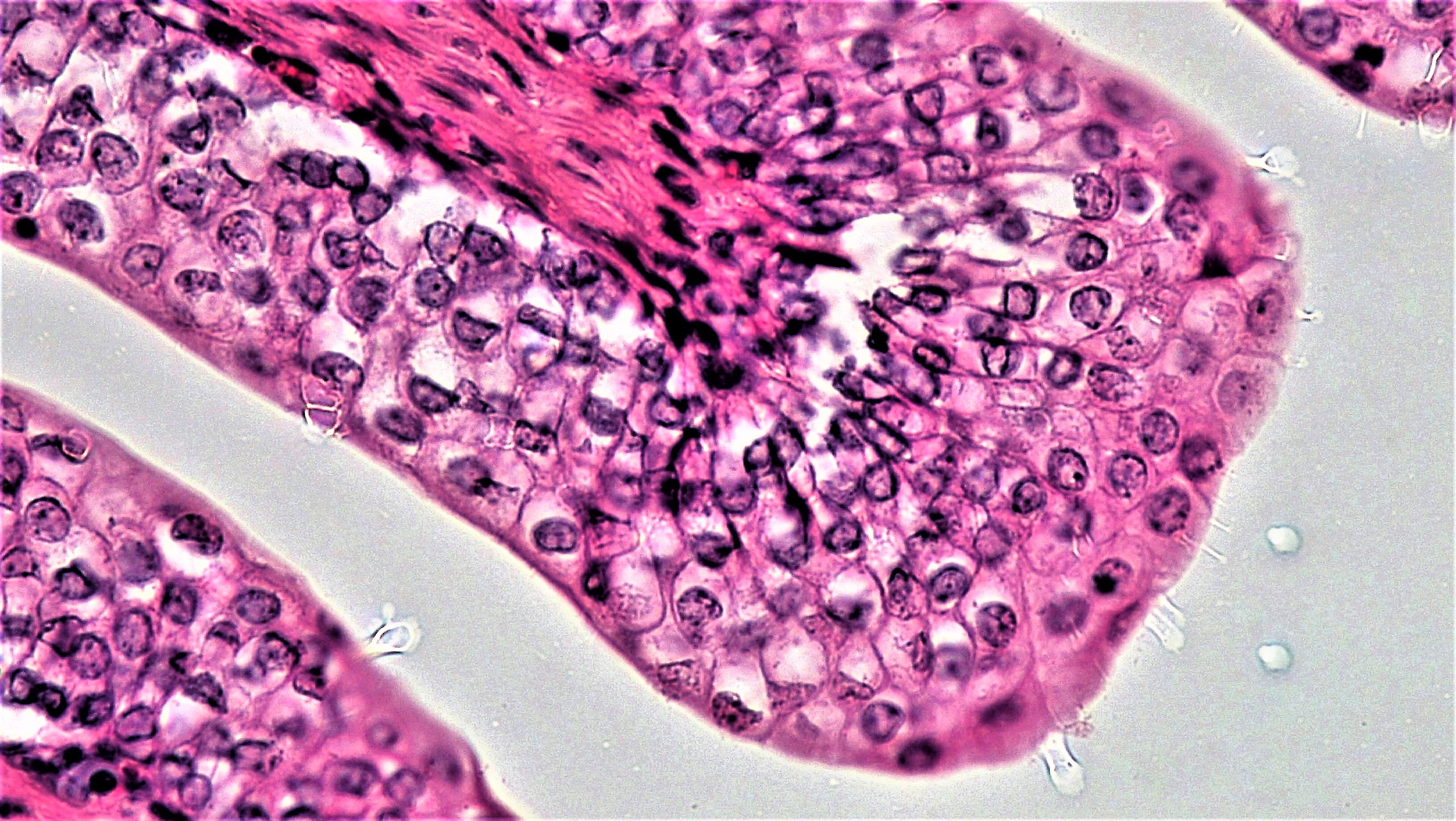
Chen, Y.-T. et al. Lineage tracing reveals distinctive fates for mesothelial cells and submesothelial fibroblasts during peritoneal injury. J. Am. Soc. Nephrol. 25, 2847–2858 (2014).
CAS
PubMed
PubMed Central
Article
Google Scholar
Lua, I., Li, Y., Pappoe, L. S. & Asahina, K. Myofibroblastic conversion and regeneration of mesothelial cells in peritoneal and liver fibrosis. Am. J. Pathol. 185, 3258–3273 (2015).
CAS
PubMed
PubMed Central
Article
Google Scholar
Ghellai, A. M. et al. Role of transforming growth factor beta-1 in peritonitis-induced adhesions. J. Gastrointest. Surg. 4, 316–323 (2000).
CAS
PubMed
Article
Google Scholar
Fraser, D., Wakefield, L. & Phillips, A. Independent regulation of transforming growth factor-beta1 transcription and translation by glucose and platelet-derived growth factor. Am. J. Pathol. 161, 1039–1049 (2002).
CAS
PubMed
PubMed Central
Article
Google Scholar
Margetts, P. J. et al. Transient overexpression of TGF-{beta}1 induces epithelial mesenchymal transition in the rodent peritoneum. J. Am. Soc. Nephrol. 16, 425–436 (2005).
CAS
PubMed
Article
Google Scholar
Loureiro, J. et al. Blocking TGF-β1 protects the peritoneal membrane from dialysate-induced damage. J. Am. Soc. Nephrol. 22, 1682–1695 (2011).
CAS
PubMed
PubMed Central
Article
Google Scholar
Patel, P. et al. Smad3-dependent and -independent pathways are involved in peritoneal membrane injury. Kidney Int. 77, 319–328 (2010).
CAS
PubMed
Article
Google Scholar
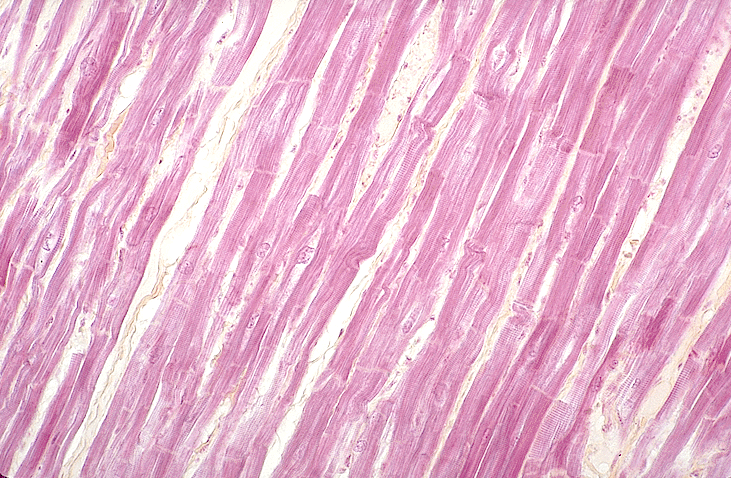
Tsai, J. M. et al. Adhesions are derived from hypoxia responsive MSLN+ mesothelial cells and are resolved via targeted therapies. Sci. Transl. Med. (2018). In the Press.
Law, M. R., Hodson, M. E. & Heard, B. E. Malignant mesothelioma of the pleura: relation between histological type and clinical behaviour. Thorax 37, 810–815 (1982).
CAS
PubMed
PubMed Central
Article
Google Scholar
Rudd, R. M. Malignant mesothelioma. Br. Med. Bull. 93, 105–123 (2010).
CAS
PubMed
Article
Google Scholar
Soeberg, M. J. et al. Incidence and survival trends for malignant pleural and peritoneal mesothelioma, Australia, 1982-2009. Occup. Environ. Med. 73, 187–194 (2016).
PubMed
Article
Google Scholar
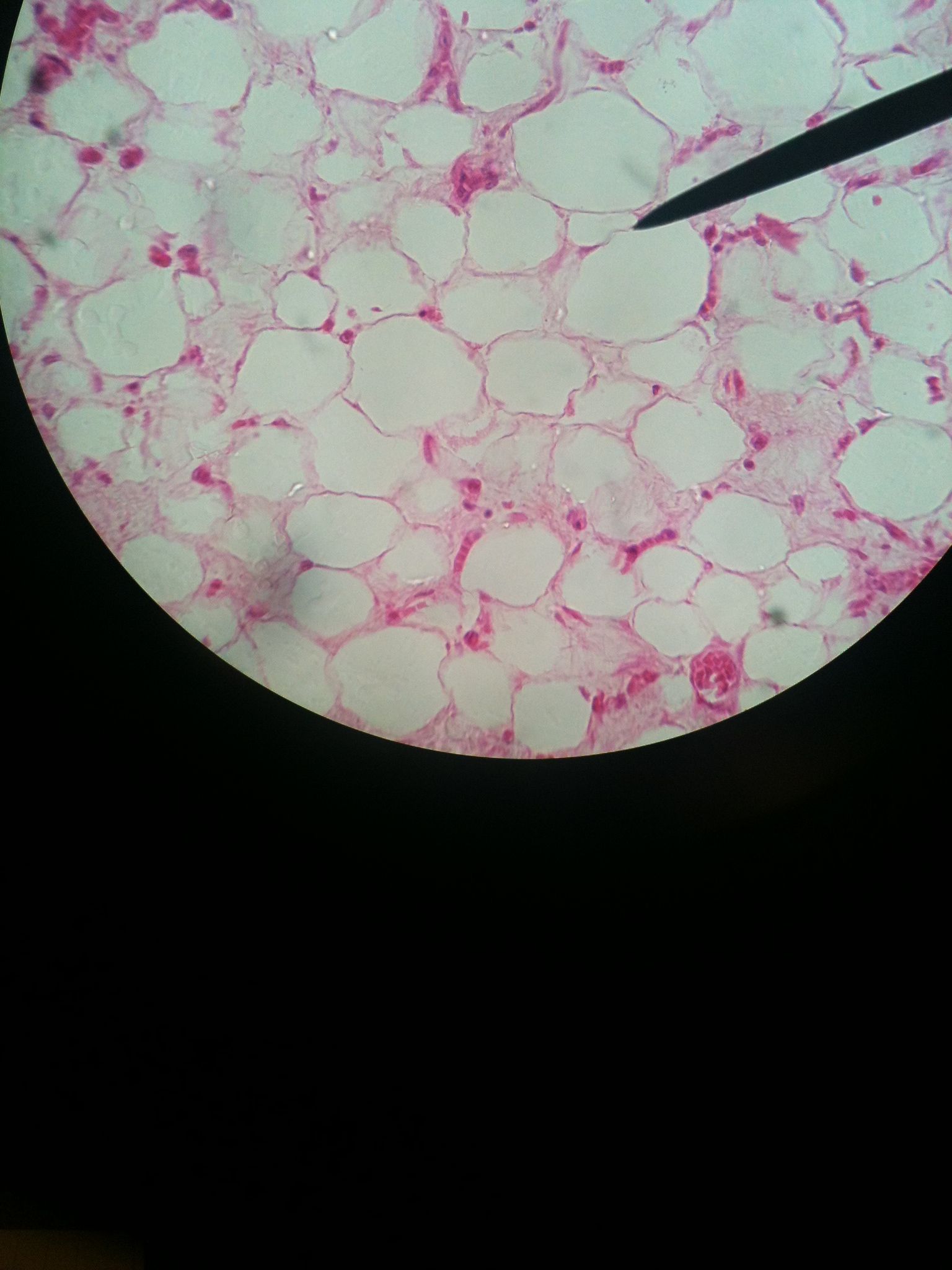
Baumann, F., Ambrosi, J.-P. & Carbone, M. Asbestos is not just asbestos: an unrecognised health hazard. Lancet Oncol. 14, 576–578 (2013).
PubMed
Article
Google Scholar
Carbone, M. et al. Combined genetic and genealogic studies uncover a large BAP1 cancer syndrome kindred tracing back nine generations to a common ancestor from the 1700s. PLoS Genet. 11, e1005633 (2015).
PubMed
PubMed Central
Article
CAS
Google Scholar
Testa, J. R. et al. Germline BAP1 mutations predispose to malignant mesothelioma. Nat. Genet. 43, 1022–1025 (2011).
CAS
PubMed
PubMed Central
Article
Google Scholar
Napolitano, A. et al. Minimal asbestos exposure in germline BAP1 heterozygous mice is associated with deregulated inflammatory response and increased risk of mesothelioma. Oncogene 35, 1996–2002 (2016).
CAS
PubMed
Article
Google Scholar
van Zandwijk, N. et al. Guidelines for the diagnosis and treatment of malignant pleural mesothelioma. J. Thorac. Dis. 5, E254–E307 (2013).
PubMed
PubMed Central
Google Scholar
Kurman, R. J. & Shih, I.-M. The origin and pathogenesis of epithelial ovarian cancer: a proposed unifying theory. Am. J. Surg. Pathol. 34, 433–443 (2010).
PubMed
PubMed Central
Article
Google Scholar
Piek, J. M. et al. Dysplastic changes in prophylactically removed Fallopian tubes of women predisposed to developing ovarian cancer. J. Pathol. 195, 451–456 (2001).
CAS
PubMed
Article
Google Scholar
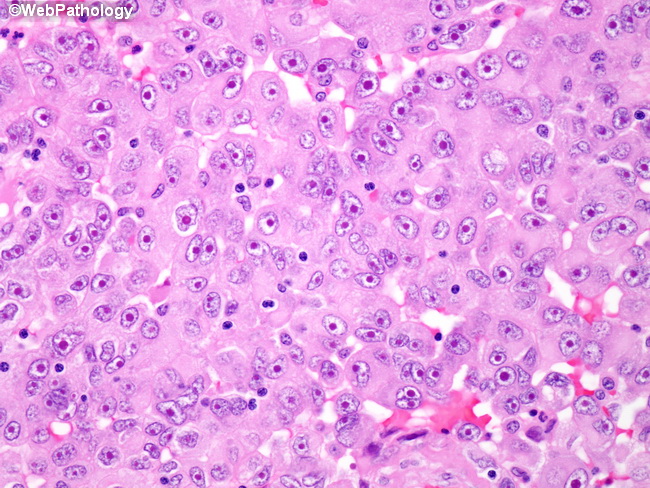
Callahan, M. J. et al. Primary fallopian tube malignancies in BRCA-positive women undergoing surgery for ovarian cancer risk reduction. J. Clin. Oncol. 25, 3985–3990 (2007).
PubMed
Article
Google Scholar
Kindelberger, D. W. et al. Intraepithelial carcinoma of the fimbria and pelvic serous carcinoma: evidence for a causal relationship. Am. J. Surg. Pathol. 31, 161–169 (2007).
PubMed
Article
Google Scholar
Eckert, M. A. et al. Genomics of ovarian cancer progression reveals diverse metastatic trajectories including intraepithelial metastasis to the fallopian tube. Cancer Discov. 6, 1342–1351 (2016).
CAS
PubMed
PubMed Central
Article
Google Scholar
Coscia, F. et al. Integrative proteomic profiling of ovarian cancer cell lines reveals precursor cell associated proteins and functional status. Nat. Commun. 7, 12645 (2016).
CAS
PubMed
PubMed Central
Article
Google Scholar
Zhang, X. Y. et al. Characteristics and growth patterns of human peritoneal mesothelial cells: comparison between advanced epithelial ovarian cancer and non-ovarian cancer sources. J. Soc. Gynecol. Investig. 6, 333–340 (1999).
CAS
PubMed
Article
Google Scholar
Chang, K. & Pastan, I. Molecular cloning of mesothelin, a differentiation antigen present on mesothelium, mesotheliomas, and ovarian cancers. Proc. Natl Acad. Sci. USA 93, 136–140 (1996).
CAS
PubMed
Article
Google Scholar
Cancer Genome Atlas Research Network. Integrated genomic analyses of ovarian carcinoma. Nature 474, 609–615 (2011).
Article
CAS
Google Scholar
-NBP2-27996-img0002.jpg)
He, X. et al. Mesothelin promotes epithelial-to-mesenchymal transition and tumorigenicity of human lung cancer and mesothelioma cells. Mol. Cancer 16, 63 (2017).
PubMed
PubMed Central
Article
CAS
Google Scholar
Bueno, R. et al. Comprehensive genomic analysis of malignant pleural mesothelioma identifies recurrent mutations, gene fusions and splicing alterations. Nat. Genet. 47, 407 (2015).
Google Scholar
de Reyniès, A. et al. Molecular classification of malignant pleural mesothelioma: identification of a poor prognosis subgroup linked to the epithelial-to-mesenchymal transition. Clin. Cancer Res. 20, 1323–1334 (2014).
PubMed
Article
CAS
Google Scholar
Bozzi, F. et al. Epithelioid peritoneal mesothelioma: a hybrid phenotype within a mesenchymal-epithelial/epithelial-mesenchymal transition framework. Oncotarget 7, 75503–75517 (2016).
PubMed
PubMed Central
Article
Google Scholar
Tan, T. Z. et al. Epithelial-mesenchymal transition spectrum quantification and its efficacy in deciphering survival and drug responses of cancer patients. EMBO Mol. Med. 6, 1279–1293 (2014).
CAS
PubMed
PubMed Central
Article
Google Scholar
Fischer, K. R. et al. Epithelial-to-mesenchymal transition is not required for lung metastasis but contributes to chemoresistance. Nature 527, 472–476 (2015).
CAS
PubMed
PubMed Central
Article
Google Scholar
Zheng, X. et al. Epithelial-to-mesenchymal transition is dispensable for metastasis but induces chemoresistance in pancreatic cancer. Nature 527, 525–530 (2015).
CAS
PubMed
PubMed Central
Article
Google Scholar

Granot, D. et al. In vivo imaging of the systemic recruitment of fibroblasts to the angiogenic rim of ovarian carcinoma tumors. Cancer Res. 67, 9180–9189 (2007).
CAS
PubMed
PubMed Central
Article
Google Scholar
Schauer, I. G., Sood, A. K., Mok, S. & Liu, J. Cancer-associated fibroblasts and their putative role in potentiating the initiation and development of epithelial ovarian cancer. Neoplasia 13, 393–405 (2011).
CAS
PubMed
PubMed Central
Article
Google Scholar
Rynne-Vidal, A. et al. Mesothelial-to-mesenchymal transition as a possible therapeutic target in peritoneal metastasis of ovarian cancer. J. Pathol. 242, 140–151 (2017).
CAS
PubMed
PubMed Central
Article
Google Scholar
Lv, Z.-D. et al. Mesothelial cells differentiate into fibroblast-like cells under the scirrhous gastric cancer microenvironment and promote peritoneal carcinomatosis in vitro and in vivo. Mol. Cell Biochem. 377, 177–185 (2013).
CAS
PubMed
Article
Google Scholar
Sandoval, P. et al. Carcinoma-associated fibroblasts derive from mesothelial cells via mesothelial-to-mesenchymal transition in peritoneal metastasis. J. Pathol. 231, 517–531 (2013).
CAS
PubMed
Article
Google Scholar
Matte, I. et al. Ovarian cancer ascites enhance the migration of patient-derived peritoneal mesothelial cells via cMet pathway through HGF-dependent and -independent mechanisms. Int. J. Cancer 137, 289–298 (2015).
CAS
PubMed
Article
Google Scholar
Satoyoshi, R. , Aiba, N., Yanagihara, K., Yashiro, M. & Tanaka, M. Tks5 activation in mesothelial cells creates invasion front of peritoneal carcinomatosis. Oncogene 34, 3176–3187 (2015).
CAS
PubMed
Article
Google Scholar
Tanaka, M. et al. Mesothelial cells create a novel tissue niche that facilitates gastric cancer invasion. Cancer Res. 77, 684–695 (2017).
CAS
PubMed
Article
Google Scholar
Nakamura, K. et al. Exosomes promote ovarian cancer cell invasion through transfer of CD44 to peritoneal mesothelial cells. Mol. Cancer Res. 15, 78–92 (2017).
CAS
PubMed
Article
Google Scholar
Greening, D. W. et al. Secreted primary human malignant mesothelioma exosome signature reflects oncogenic cargo. Sci. Rep. 6, 32643 (2016).
CAS
PubMed
PubMed Central
Article
Google Scholar
Yokoi, A. et al. Malignant extracellular vesicles carrying MMP1 mRNA facilitate peritoneal dissemination in ovarian cancer. Nat. Commun. 8, 14470 (2017).
PubMed
PubMed Central
Article
Google Scholar
Wei, M. et al. Malignant ascites-derived exosomes promote proliferation and induce carcinoma-associated fibroblasts transition in peritoneal mesothelial cells. Oncotarget 8, 42262–42271 (2017).
PubMed
PubMed Central
Google Scholar
Deng, G. et al. Gastric cancer-derived exosomes promote peritoneal metastasis by destroying the mesothelial barrier. FEBS Lett. 591, 2167–2179 (2017).
CAS
PubMed
Article
Google Scholar

Huang, R. Y.-J. et al. An EMT spectrum defines an anoikis-resistant and spheroidogenic intermediate mesenchymal state that is sensitive to e-cadherin restoration by a src-kinase inhibitor, saracatinib (AZD0530). Cell Death Dis. 4, e915 (2013).
CAS
PubMed
PubMed Central
Article
Google Scholar
Verhaak, R. G. W. et al. Prognostically relevant gene signatures of high-grade serous ovarian carcinoma. J. Clin. Invest. 123, 517–525 (2013).
CAS
PubMed
Google Scholar
Chu, Y.-S. et al. Prototypical type I E-cadherin and type II cadherin-7 mediate very distinct adhesiveness through their extracellular domains. J. Biol. Chem. 281, 2901–2910 (2006).
CAS
PubMed
Article
Google Scholar
Hohenstein, P. & Hastie, N. D. The many facets of the Wilms’ tumour gene, WT1. Hum. Mol. Genet. 15(Spec No 2), R196–R201 (2006).
CAS
PubMed
Article
Google Scholar
Bradford, S. T. et al. A cell-autonomous role for WT1 in regulating Sry in vivo. Hum. Mol. Genet. 18, 3429–3438 (2009).
CAS
PubMed
Article
Google Scholar
Carmona, R. et al. Conditional deletion of WT1 in the septum transversum mesenchyme causes congenital diaphragmatic hernia in mice. eLife 5, e16009 (2016).
Essafi, A. et al. A wt1-controlled chromatin switching mechanism underpins tissue-specific wnt4 activation and repression. Dev. Cell. 21, 559–574 (2011).
CAS
PubMed
PubMed Central
Article
Google Scholar
Tallini, Y. N. et al. c-kit expression identifies cardiovascular precursors in the neonatal heart. Proc. Natl Acad. Sci. USA 106, 1808–1813 (2009).
CAS
PubMed
Article
Google Scholar
van Tuyn, J. et al. Epicardial cells of human adults can undergo an epithelial-to-mesenchymal transition and obtain characteristics of smooth muscle cells in vitro. Stem Cells 25, 271–278 (2007).
PubMed
Article
CAS
Google Scholar
Chong, J. J. H. et al. Adult cardiac-resident MSC-like stem cells with a proepicardial origin. Cell Stem Cell 9, 527–540 (2011).
CAS
PubMed
PubMed Central
Article
Google Scholar
Cabezas-Wallscheid, N. et al. Vitamin A-retinoic acid signaling regulates hematopoietic stem cell dormancy. Cell 169, 807–823.e19 (2017).
CAS
PubMed
Article
Google Scholar

Bollini, S. et al. Re-activated adult epicardial progenitor cells are a heterogeneous population molecularly distinct from their embryonic counterparts. Stem. Cells Dev. 23, 1719–1730 (2014).
CAS
PubMed
Article
Google Scholar
Ziv, O., Glaser, B. & Dor, Y. The plastic pancreas. Dev. Cell 26, 3–7 (2013).
CAS
PubMed
Article
Google Scholar
Pan, F. C. et al. Spatiotemporal patterns of multipotentiality in Ptf1a-expressing cells during pancreas organogenesis and injury-induced facultative restoration. Development 140, 751–764 (2013).
CAS
PubMed
PubMed Central
Article
Google Scholar
Hassan, R. et al. Anti-tumor activity of K1-LysPE38QQR, an immunotoxin targeting mesothelin, a cell-surface antigen overexpressed in ovarian cancer and malignant mesothelioma. J. Immunother. 23, 473–479 (2000).
CAS
PubMed
Article
Google Scholar
Hung, C.-F., Calizo, R., Tsai, Y.-C., He, L. & Wu, T.-C. A DNA vaccine encoding a single-chain trimer of HLA-A2 linked to human mesothelin peptide generates anti-tumor effects against human mesothelin-expressing tumors. Vaccine 25, 127–135 (2007).
CAS
PubMed
Article
Google Scholar
Tang, Z. et al. A human single-domain antibody elicits potent antitumor activity by targeting an epitope in mesothelin close to the cancer cell surface. Mol. Cancer Ther. 12, 416–426 (2013).
CAS
PubMed
PubMed Central
Article
Google Scholar
Hassan, R. et al. Preclinical evaluation of MORAb-009, a chimeric antibody targeting tumor-associated mesothelin. Cancer Immun. 7, 20 (2007).
PubMed
PubMed Central
Google Scholar
Hassan, R. et al. Phase II clinical trial of amatuximab, a chimeric antimesothelin antibody with pemetrexed and cisplatin in advanced unresectable pleural mesothelioma. Clin. Cancer Res. 20, 5927–5936 (2014).
CAS
PubMed
PubMed Central
Article
Google Scholar
Silva, I. A. et al. Aldehyde dehydrogenase in combination with CD133 defines angiogenic ovarian cancer stem cells that portend poor patient survival. Cancer Res. 71, 3991–4001 (2011).
CAS
PubMed
PubMed Central
Article
Google Scholar
Cortes-Dericks, L., Froment, L., Boesch, R., Schmid, R. A. & Karoubi, G. Cisplatin-resistant cells in malignant pleural mesothelioma cell lines show ALDH(high)CD44(+) phenotype and sphere-forming capacity. BMC Cancer 14, 304 (2014).
PubMed
PubMed Central
Article
CAS
Google Scholar
Bodnar, L. et al. Wnt/β-catenin pathway as a potential prognostic and predictive marker in patients with advanced ovarian cancer. J. Ovarian Res. 7, 16 (2014).
PubMed
PubMed Central
Article
CAS
Google Scholar
Heldin, P. & Pertoft, H. Synthesis and assembly of the hyaluronan-containing coats around normal human mesothelial cells. Exp. Cell Res. 208, 422–429 (1993).
CAS
PubMed
Article
Google Scholar
Yung, S. et al. Source of peritoneal proteoglycans. Human peritoneal mesothelial cells synthesize and secrete mainly small dermatan sulfate proteoglycans. Am. J. Pathol. 146, 520–529 (1995).
CAS
PubMed
PubMed Central
Google Scholar
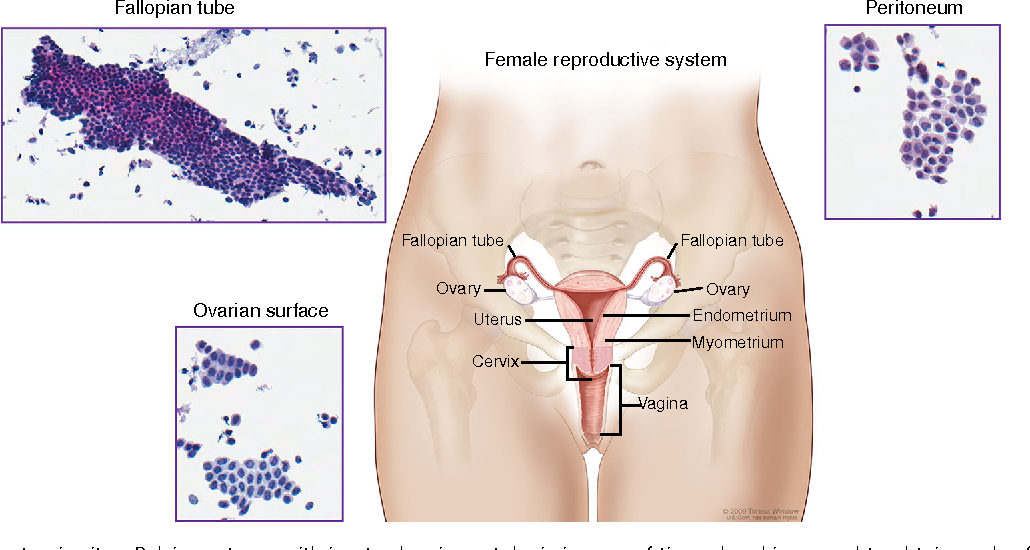
Bellingan, G. J., Caldwell, H., Howie, S. E., Dransfield, I. & Haslett, C. In vivo fate of the inflammatory macrophage during the resolution of inflammation: inflammatory macrophages do not die locally, but emigrate to the draining lymph nodes. J. Immunol. 157, 2577–2585 (1996).
CAS
PubMed
Google Scholar
Bellingan, G. J. et al. Adhesion molecule-dependent mechanisms regulate the rate of macrophage clearance during the resolution of peritoneal inflammation. J. Exp. Med. 196, 1515–1521 (2002).
CAS
PubMed
PubMed Central
Article
Google Scholar
Gosselin, D. et al. Environment drives selection and function of enhancers controlling tissue-specific macrophage identities. Cell 159, 1327–1340 (2014).
CAS
PubMed
PubMed Central
Article
Google Scholar
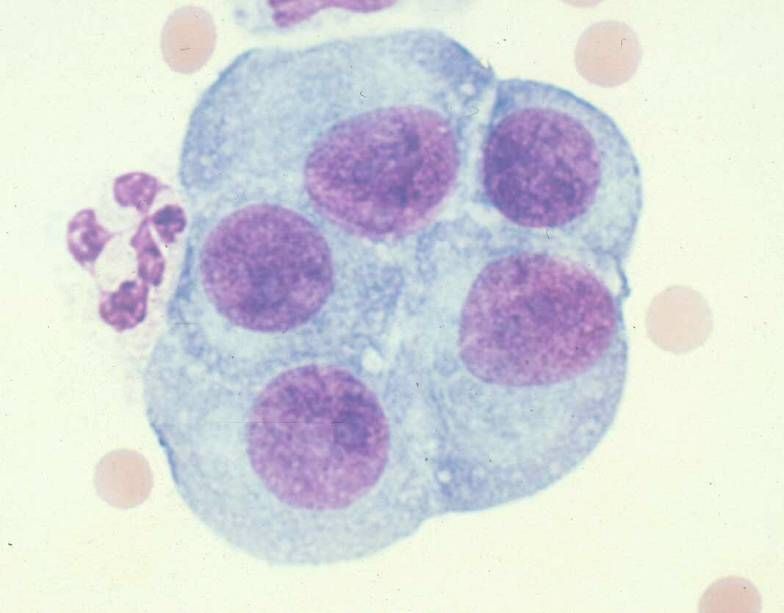
Lavin, Y. et al. Tissue-resident macrophage enhancer landscapes are shaped by the local microenvironment. Cell 159, 1312–1326 (2014).
CAS
PubMed
PubMed Central
Article
Google Scholar
Davies, L. C. et al. Peritoneal tissue-resident macrophages are metabolically poised to engage microbes using tissue-niche fuels. Nat. Commun. 8, 2074 (2017).
PubMed
PubMed Central
Article
CAS
Google Scholar
Avraham-Chakim, L. et al. Fluid-flow induced wall shear stress and epithelial ovarian cancer peritoneal spreading. PLoS ONE 8, e60965 (2013).
CAS
PubMed
PubMed Central
Article
Google Scholar
Weber, G. F. et al. Pleural innate response activator B cells protect against pneumonia via a GM-CSF-IgM axis. J. Exp. Med. 211, 1243–1256 (2014).
CAS
PubMed
PubMed Central
Article
Google Scholar
Wang, J. & Kubes, P. A reservoir of mature cavity macrophages that can rapidly invade visceral organs to affect tissue repair. Cell 165, 668–678 (2016).
CAS
PubMed
Article
Google Scholar
Yung, S. & Chan, T. M. Intrinsic cells: mesothelial cells—central players in regulating inflammation and resolution. Perit. Dial. Int. 29(Suppl 2), S21–S27 (2009).
CAS
PubMed
Google Scholar
Rangel-Moreno, J. et al. Omental milky spots develop in the absence of lymphoid tissue-inducer cells and support B and T cell responses to peritoneal antigens. Immunity 30, 731–743 (2009).
CAS
PubMed
PubMed Central
Article
Google Scholar
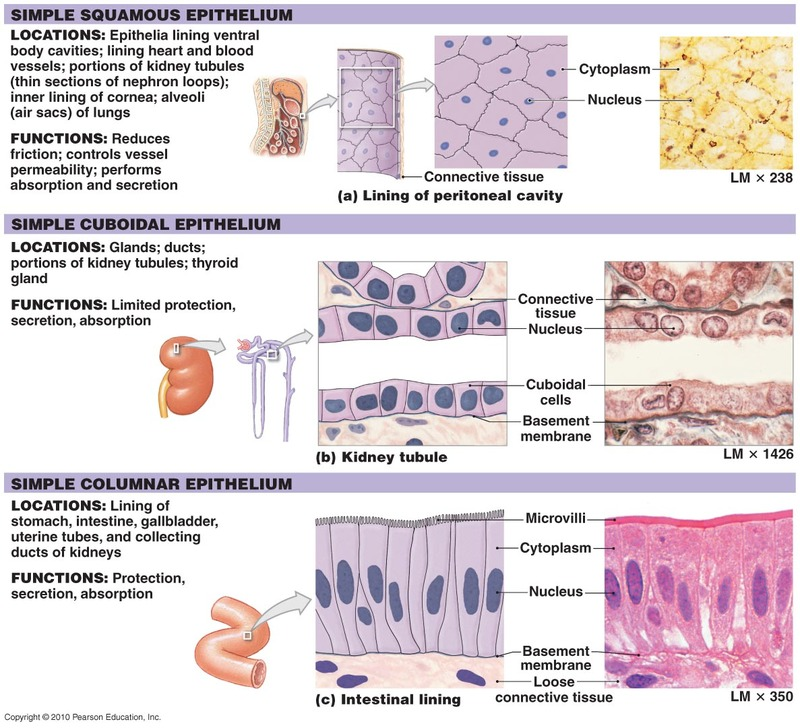
Van Vugt, E., Van Rijthoven, E. A., Kamperdijk, E. W. & Beelen, R. H. Omental milky spots in the local immune response in the peritoneal cavity of rats. Anat. Rec. 244, 235–245 (1996).
PubMed
Article
Google Scholar
Ansel, K. M., Harris, R. B. S. & Cyster, J. G. CXCL13 is required for B1 cell homing, natural antibody production, and body cavity immunity. Immunity 16, 67–76 (2002).
CAS
PubMed
Article
Google Scholar
What Is Malignant Mesothelioma?
Malignant mesothelioma is cancer that starts in cells in the linings of certain parts of the body, most commonly the linings of the chest or abdomen (belly).
Cancer starts when cells start to grow out of control. Cells in nearly any part of the body can become cancer. To learn more about how cancers start and spread, see What Is Cancer?
The mesothelium
A layer of specialized cells called mesothelial cells lines the inside of your chest, your abdomen, and the space around your heart. These cells also cover the outer surface of most of your internal organs. The lining formed by these cells is called the mesothelium.
The mesothelium helps protect your organs by making a special lubricating fluid that allows organs to slide against each other. For instance, this fluid makes it easier for your lungs to move (expand and contract) inside your chest when you breathe. The mesothelium has different names in different parts of the body:
- The pleura covers the lungs and the space in the chest that contains the lungs.
- The peritoneum lines the inside of the abdomen and covers many of the organs in the abdomen.
- The pericardium covers the heart and the space that holds the heart in the chest.
- The tunica vaginalis lines the testicles.
Types of malignant mesothelioma
Mesothelial tumors can start in any of these linings. These tumors can be cancer (malignant) or not cancer (benign).
A cancer tumor of the mesothelium is called a malignant mesothelioma. This is often shortened to just mesothelioma. Mesotheliomas can start in 4 main parts of the body.
- Pleural mesotheliomas start in the chest. More
than 3 out of 4 mesotheliomas are pleural mesotheliomas. - Peritoneal mesotheliomas start in the abdomen. They make up most of the remaining cases.
- Pericardial mesotheliomas start in the covering around the heart and are very rare.
- Mesotheliomas of the tunica vaginalis are very rare tumors that start in the covering layer of the testicles.
Malignant mesotheliomas are grouped into 3 main
types based on how the cancer cells look:
- More than half of mesotheliomas are epithelioid. This type tends to have a better outlook (prognosis) than the other types.
- About 10% to 20% of mesotheliomas are sarcomatoid (fibrous).
- Mixed (biphasic) mesotheliomas have both epithelioid and sarcomatoid areas. They make up the remaining 20% to 30% of mesotheliomas.
Metamorphosis of mesothelial cells with active horizontal motility in tissue culture
Mutsaers, S. E. Mesothelial cells: their structure, function and role in serosal repair. Respirology 7, 171–191 (2002).
Article
Google Scholar
Herrick, S. E. & Mutsaers, S. E. Mesothelial progenitor cells and their potential in tissue engineering. Int. J. Biochem. Cell Biol. 36, 621–642 (2004).
CAS
Article
Google Scholar
Andrews, P. M. & Porter, K. R. The ultrastructural morphology and possible functional significance of mesothelial microvilli. Anat. Rec. 177, 409–426 (1973).
CAS
Article
Google Scholar
Fedorko, M. E. & Hirsch, J. G. Studies on transport of macromolecules and small particles across mesothelial cells of the mouse omentum. I. Morphologic aspects. Exp. Cell Res. 69, 113–127 (1971).
CAS
Article
Google Scholar
Yung, S. & Chan, T. M. Intrinsic cells: mesothelial cells – central players in regulating inflammation and resolution. Perit. Dial. Int. 29 Suppl 2, S21–27 (2009).
PubMed
Google Scholar
Van Der Wal, B. C., Hofland, L. J. Marquet, R. L., Van Koetsveld, P. M., Van Rossen, M. E. & Van Eijck, C. H. Paracrine interactions between mesothelial and colon-carcinoma cells in a rat model. Int. J. Cancer 73, 885–890 (1997).
CAS
Article
Google Scholar
Hu, Q., Akatsuka, S., Yamashita, Y., Ohara, H., Nagai, H., Okazaki, Y., Takahashi, T. & Toyokuni, S. Homozygous deletion of CDKN2A/2B is a hallmark of iron-induced high-grade rat mesothelioma. Lab. Invest. 90, 360–373 (2010).
CAS
Article
Google Scholar
Nagai, H., Okazaki, Y., Chew, S. H., Misawa, N., Yamashita, Y., Akatsuka, S., Ishihara, T., Yamashita, K., Yoshikawa, Y., Yasui, H., Jiang, L., Ohara, H., Takahashi, T., Ichihara, G., Kostarelos, K., Miyata, Y., Shinohara, H. & Toyokuni, S. Diameter and rigidity of multiwalled carbon nanotubes are critical factors in mesothelial injury and carcinogenesis. Proc. Natl. Acad. Sci. USA 108, E1330–1338 (2011).
Article
Google Scholar
Akatsuka, S., Yamashita, Y., Ohara, H., Liu, Y. T., Izumiya, M., Abe, K., Ochiai, M., Jiang, L., Nagai, H., Okazaki, Y., Murakami, H., Sekido, Y., Arai, E., Kanai, Y., Hino, O., Takahashi, T., Nakagama, H. & Toyokuni, S. Fenton reaction induced cancer in wild type rats recapitulates genomic alterations observed in human cancer. PLoS One 7, e43403 (2012).
ADS
CAS
Article
Google Scholar
Jiang, L., Akatsuka, S., Nagai, H., Chew, S. H., Ohara, H., Okazaki, Y., Yamashita, Y., Yoshikawa, Y., Yasui, H., Ikuta, K., Sasaki, K., Kohgo, Y., Hirano, S., Shinohara, Y., Kohyama, N., Takahashi, T. & Toyokuni, S. Iron overload signature in chrysotile-induced malignant mesothelioma. J. Pathol. 228, 366–377 (2012).
CAS
Article
Google Scholar
Nagai, H. & Toyokuni, S. Differences and similarities between carbon nanotubes and asbestos fibers during mesothelial carcinogenesis: shedding light on fiber entry mechanism. Cancer Sci. 103, 1378–1390 (2012).
CAS
Article
Google Scholar
Dobbie, J. W., Zaki, M. & Wilson, L. Ultrastructural studies on the peritoneum with special reference to chronic ambulatory peritoneal dialysis. Scott. Med. J. 26, 213–223 (1981).
CAS
Article
Google Scholar
Di Paolo, S., Gesualdo, L., Ranieri, E., Grandaliano, G. & Schena, F. P. High glucose concentration induces the overexpression of transforming growth factor-beta through the activation of a platelet-derived growth factor loop in human mesangial cells. Am. J. Pathol. 149, 2095–2106 (1996).
CAS
PubMed
PubMed Central
Google Scholar
Ellis, H. The cause and prevention of postoperative intraperitoneal adhesions. Surg. Gynecol. Obstet. 133, 497–511 (1971).
CAS
PubMed
Google Scholar
Nagai, H. & Toyokuni, S. Biopersistent fiber-induced inflammation and carcinogenesis: lessons learned from asbestos toward safety of fibrous nanomaterials. Arch. Biochem. Biophys. 502, 1–7 (2010).
CAS
Article
Google Scholar
Mutsaers, S. E., Whitaker, D. & Papadimitriou, J. M. Mesothelial regeneration is not dependent on subserosal cells. J. Pathol. 190, 86–92 (2000).
CAS
Article
Google Scholar
Whitaker, D. & Papadimitriou, J. Mesothelial healing: morphological and kinetic investigations. J. Pathol. 145, 159–175 (1985).
CAS
Article
Google Scholar
Ryan, G. B., Grobety, J. & Majno, G. Mesothelial injury and recovery. Am. J. Pathol. 71, 93–112 (1973).
CAS
PubMed
PubMed Central
Google Scholar
Foley-Comer, A. J., Herrick, S. E., Al-Mishlab, T., Prele, C. M., Laurent, G. J. & Mutsaers, S. E. Evidence for incorporation of free-floating mesothelial cells as a mechanism of serosal healing. J. Cell Sci. 115, 1383–1389 (2002).
CAS
PubMed
Google Scholar
Bolen, J. W., Hammar, S. P. & Mcnutt, M. A. Reactive and neoplastic serosal tissue. A light-microscopic, ultrastructural and immunocytochemical study. Am. J. Surg. Pathol. 10, 34–47 (1986).
CAS
Article
Google Scholar
Pampinella, F., Roelofs, M., Castellucci, E., Chiavegato, A., Guidolin, D., Passerini-Glazel, G., Pagano, F. & Sartore, S. Proliferation of submesothelial mesenchymal cells during early phase of serosal thickening in the rabbit bladder is accompanied by transient keratin 18 expression. Exp. Cell Res. 223, 327–339 (1996).
CAS
Article
Google Scholar
Mutsaers, S. E., Whitaker, D. & Papadimitriou, J. M. Stimulation of mesothelial cell proliferation by exudate macrophages enhances serosal wound healing in a murine model. Am. J. Pathol. 160, 681–692 (2002).
Article
Google Scholar
Fotev, Z., Whitaker, D. & Papadimitriou, J. M. Role of macrophages in mesothelial healing. J. Pathol. 151, 209–219 (1987).
CAS
Article
Google Scholar
Mutsaers, S. E. The mesothelial cell. Int. J. Biochem. Cell Biol. 36, 9–16 (2004).
CAS
Article
Google Scholar
Yap, A. S. & Kovacs, E. M. Direct cadherin-activated cell signaling: a view from the plasma membrane. J. Cell Biol. 160, 11–16 (2003).
CAS
Article
Google Scholar
Hazan, R. B., Qiao, R., Keren, R., Badano, I. & Suyama, K. Cadherin switch in tumor progression. Ann. N. Y. Acad. Sci. 1014, 155–163 (2004).
ADS
CAS
Article
Google Scholar
Hott, J. W., Sparks, J. A., Godbey, S. W. & Antony, V. B. Mesothelial cell response to pleural injury: thrombin-induced proliferation and chemotaxis of rat pleural mesothelial cells. Am. J. Respir. Cell Mol. Biol. 6, 421–425 (1992).
CAS
Article
Google Scholar
Nasreen, N., Mohammed, K. A., Galffy, G., Ward, M. J. & Antony, V. B. MCP-1 in pleural injury: CCR2 mediates haptotaxis of pleural mesothelial cells. Am. J. Physiol. -Lung Cell. Mol. Physiol. 278, L591–598 (2000).
CAS
Article
Google Scholar
Strukova, S. M. Thrombin as a regulator of inflammation and reparative processes in tissues. Biochemistry (Mosc). 66, 8–18 (2001).
CAS
Article
Google Scholar
Shireman, P. K., Contreras-Shannon, V., Ochoa, O., Karia, B. P., Michalek, J. E. & Mcmanus, L. M. MCP-1 deficiency causes altered inflammation with impaired skeletal muscle regeneration. J. Leukoc. Biol. 81, 775–785 (2007).
CAS
Article
Google Scholar
Topley, N., Brown, Z., Jorres, A., Westwick, J., Davies, M., Coles G.A. & Williams J.D. Human peritoneal mesothelial cells synthesize interleukin-8. Synergistic induction by interleukin-1 beta and tumor necrosis factor-alpha. Am. J. Pathol. 142, 1876–1886 (1993).
CAS
PubMed
PubMed Central
Google Scholar
Topley, N., Jorres, A., Luttmann, W., Petersen, M. M., Lang, M. J. Thierauch, K. H., Muller, C., Coles, G. A., Davies, M. & Williams, J. D. Human peritoneal mesothelial cells synthesize interleukin-6: induction by IL-1 beta and TNF alpha. Kidney Int. 43, 226–233 (1993).
CAS
Article
Google Scholar
Eigenbrod, T., Park, J. H., Harder, J., Iwakura, Y. & Nunez, G. Cutting edge: critical role for mesothelial cells in necrosis-induced inflammation through the recognition of IL-1 alpha released from dying cells. J. Immunol. 181, 8194–8198 (2008).
CAS
Article
Google Scholar
history, challenges and future directions
Mesothelial cells line the surface of the pleura, pericardium, peritoneum and internal reproductive organs. One of their main functions is to act as a non-adhesive barrier to protect against physical damage, however, over the past decades their physiological and pathological properties have been revealed in association with a variety of conditions and diseases. Mesothelium has been used in surgical operations in clinical settings, such as omental patching for perforated peptic ulcers and in glutaraldehyde-treated autologous pericardium for aortic valve reconstruction. Various methods for mesothelial cell transplantation have also been established and developed, particularly within the area of tissue engineering, including scaffold and non-scaffold cell sheet technologies. However, the use of mesothelial cell transplantation in patients remains challenging, as it requires additional operations under general anesthesia in order to obtain enough intact cells for culture. Moreover, the current methods of mesothelial cell transplantation are expensive and are not yet available in clinical practice. This review firstly summarizes the history of the use of mesothelial cell transplantation in tissue engineering, and then critically discusses the barriers for the clinical application of mesothelial cell transplantation. Finally, the recent developments in xenotransplantation technologies are discussed to evaluate other feasible alternatives to mesothelial cell transplantation.
References
1. Kawanishi K. Diverse properties of the mesothelial cells in health and disease. Pleura and Peritoneum 2016;1:79–89. Search in Google Scholar
2. Bertleff Mariëtta JO, Lange Johan F. Perforated peptic ulcer disease: a review of history and treatment. Dig Surg 2010;27:161–9. Search in Google Scholar
3. Al Halees Z, Al Shahid M, Al Sanei A, Sallehuddin A, Duran C. Up to 16 years follow-up of aortic valve reconstruction with pericardium: a stentless readily available cheap valve? Eur J Cardiothorac Surg 2005;28:200–5. Search in Google Scholar
4. Odim J, Laks H, Allada V, Child J, Wilson S, Gjertson D. Results of aortic valve-sparing and restoration with autologous pericardial leaflet extensions in congenital heart disease. Ann Thorac Surg 2005;80:647–53. Search in Google Scholar
5. Ozaki S, Kawase I, Yamashita H, Uchida S, Nozawa Y, Matsuyama T, et al. Aortic valve reconstruction using self-developed aortic valve plasty system in aortic valve disease. Interact Cardiovasc Thorac Surg 2011;2:550–3. Search in Google Scholar
6. Ozaki S, Kawase I, Yamashita H, Uchida S, Nozawa Y, Takatoh M, et al. A total of 404 cases of aortic valve reconstruction with glutaraldehyde-treated autologous pericardium. J Thorac Cardiovasc Surg 2014;147:301–6. Search in Google Scholar
7. Brown JM, O‘Brien SM, Wu C, Sikora JA, Griffith BP, Gammie JS. Isolated aortic valve replacement in North America comprising 108,687 patients in 10 years: changes in risks, valve types, and outcomes in the Society of Thoracic Surgeons National Database. J Thorac Cardiovasc Surg 2009;137:82–90. Search in Google Scholar
8. Clarke JM, Pittilo RM, Nicholson LJ, Woolf N, Seeding MA. Dacron arterial prostheses with peritoneal mesothelial cells: a preliminary morphological study. Br J Surg 1984;71:492–4. Search in Google Scholar
9. Bull HA, Pittilo RM, Drury J, Pollock JG, Clarke JM, Woolf N, et al. Effects of autologous mesothelial cell seeding on prostacyclin production within Dacron arterial prostheses. Br J Surg 1988;75:671–4. Search in Google Scholar
10. Van de Velde VJ, Herman AG, Bult H. Effects of dexamethasone on prostacyclin biosynthesis in rabbit mesothelial cells. Prostaglandins 1986;32:169–78. Search in Google Scholar
11. Di Paolo N, Sacchi G, Vanni L, Corazzi S, Pallini V, Rossi P, et al. Implant of autologous mesothelial cells in animals and a peritoneal dialysis patient. Int J Artif Organs 1989;12:485–501. Search in Google Scholar
12. Di Paolo N, Vanni L, Sacchi G. Autologous implant of peritoneal mesothelium in rabbits and man. Clin Nephrol 1990;34:179–84. Search in Google Scholar
13. Di Paolo N, Sacchi G, Vanni L, Corazzi S, Terrana B, Rossi P, et al. Autologous peritoneal mesothelial cell implant in rabbits and peritoneal dialysis patients. Nephron 1991;57:323–31. Search in Google Scholar
14. Bertram P, Tietze L, Hoopmann M, Treutner KH, Mittermayer C, Schumpelick V. Intraperitoneal transplantation of isologous mesothelial cells for prevention of adhesions. Eur J Surg 1999;165:705–9. Search in Google Scholar
15. Elmadbouh I, Chen Y, Louedec L, Silberman S, Pouzet B, Meilhac O, et al. Mesothelial cell transplantation in the infarct scar induces neovascularization and improves heart function. Cardiovasc Res 2005;68:307–17. Search in Google Scholar
16. Witkowicz J. Mesothelial cell transplantation. Pol Arch Med Wewn 2008;118:307–13. Search in Google Scholar
17. Sakaguchi K, Shimizu T, Horaguchi S, Sekine H, Yamato M, Umezu M, et al. In vitro engineering of vascularized tissue surrogates. Sci Rep 2013;3:1316. Search in Google Scholar
18. Tang Z, Okano T. Recent development of temperature-responsive surfaces and their application for cell sheet engineering. Regen Biomater 2014;1:91–102. Search in Google Scholar
19. Lachaud CC, Rodriguez-Campins B, Hmadcha A, Soria B. Use of mesothelial cells and biological matrices for tissue engineering of simple epithelium surrogates. Front Bioeng Biotechnol 2015;3:117. Search in Google Scholar
20. Esvelt Kevin M, Wang Harris H. Genome scale engineering for systems and synthetic biology. Mol Syst Biol 2013;9:641. Search in Google Scholar
21. Sander JD, Joung JK. CRISPR-cas systems for editing, regulating and targeting genomes. Nat Biotechnol 2014;32:347–55. Search in Google Scholar
22. Niemann H, Petersen B. The production of multi-transgenic pigs: update and perspectives for xenotransplantation. Transgenic Res 2016;25:361–74. Search in Google Scholar
23. Elliott RB, Escobar L, Tan PL, Muzina M, Zwain S, Buchanan C. Live encapsulated porcine islets from a type 1 diabetic patient 9.5 yr after xenotransplantation. Xenotransplantation 2007;14:157–61. Search in Google Scholar
24. Zhu HT, Wang WL, Yu L, Wang B. Pig-islet xenotransplantation: recent progress and current perspectives. Front Surg 2014;1:7. Search in Google Scholar
25. Louagie Y, Legrand-Monsieur A, Remacle C, Maldague P, Lambotte L, Ponlot R. Morphology and fibrinolytic activity of canine autogenous mesothelium used as venous substitute. Res Exp Med (Berl) 1986;186:239–47. Search in Google Scholar
26. Theuer CJ, Bergamini TM, Theuer HH, Burns CD, Proctor ML, Garrison RN. Vena cava replacement with a peritoneum-lined vascular graft. ASAIO J 1996;42:266–70. Search in Google Scholar
27. Verhagen HJ, Blankensteijn JD, de Groot PG, Heijnen-Snyder GJ, Pronk A, Vroom TM, et al. In vivo experiments with mesothelial cell seeded ePTFE vascular grafts. Eur J Vasc Endovasc Surg 1998;15:489–96. Search in Google Scholar
28. Sparks SR, Tripathy U, Broudy A, Bergan JJ, Kumins NH,Owens EL, et al. Small-caliber mesothelial cell-layered polytetraflouroethylene vascular grafts in New Zealand white rabbits. Ann Vasc Surg 2002;16:73–6. Search in Google Scholar
29. Sarkar S, Sales KM, Hamilton G, Seifalian AM. Addressing thrombogenicity in vascular graft construction. J Biomed Mater Res B Appl Biomater 2007;82:100–8. Search in Google Scholar
30. Campbell JH, Efendy JL, Campbell GR. Novel vascular graft grown within recipient’s own peritoneal cavity. Circ Res 1999;85:1173–8. Search in Google Scholar
31. Lundborg G, Dahlin LB, Danielsen N, Hansson HA, Johannesson A, Longo FM, et al. Nerve regeneration across an extended gap: a neurobiological view of nerve repair and the possible involvement of neuronotrophic factors. J Hand Surg Am 1982;7:580–7. Search in Google Scholar
32. Hekking LH, Zweers MM, Keuning ED, Driesprong BA, de Waart DR, Beelen RH, et al. Apparent successful mesothelial cell transplantation hampered by peritoneal activation. Kidney Int 2005;68:2362–7. Search in Google Scholar
33. Hotta Y, Kaneko K, Inuma J, Inami Y, Aruga S, Shimaoka T, et al. Establishment of a peritoneal mesothelial cell line from a transgenic rat harbouring the temperature-sensitive simian virus 40 large T-antigen gene. Nephrol Dial Transplant 2010;25:1825–32. Search in Google Scholar
34. Kanda R, Hamada C, Kaneko K, Nakano T, Wakabayashi K, Hara K, et al. Paracrine effects of transplanted mesothelial cells isolated from temperature-sensitive SV40 large T-antigen gene transgenic rats during peritoneal repair. Nephrol Dial Transplant 2014;29:289–300. Search in Google Scholar
35. Kitamura S, Horimoto N, Tsuji K, Inoue A, Takiue K, Sugiyama H, et al. The selection of peritoneal mesothelial cells is important for cell therapy to prevent peritoneal fibrosis. Tissue Eng Part A 2014;20:529–39. Search in Google Scholar
36. Nagy JA, Shockley TR, Masse EM, Harvey VS, Jackman RW. Mesothelial cell-mediated gene therapy: feasibility of an ex vivo strategy. Gene Ther 1995;2:393–401. Search in Google Scholar
37. Hekking LH, Harvey VS, Havenith CE, van den Born J, Beelen RH, Jackman RW, et al. Mesothelial cell transplantation in models of acute inflammation and chronic peritoneal dialysis. Perit Dial Int 2003;23:323–30. Search in Google Scholar
38. Murphy JE, Rheinwald JG. Intraperitoneal injection of genetically modified, human mesothelial cells for systemic gene therapy. Hum Gene Ther 1997;8:1867–79. Search in Google Scholar
39. Einbinder T, Sufaro Y, Yusim I, Byk G, Passlick-Deetjen J, Chaimovitz C, et al. Correction of anemia in uremic mice by genetically modified peritoneal mesothelial cells. Kidney Int 2003;63:2103–12. Search in Google Scholar
40. Bourahla B, Shafy A, Meilhac O, Elmadbouh I, Michel JB, Chachques JC. Mesothelial cells vs. skeletal myoblasts for myocardial infarction. Asian Cardiovasc Thorac Ann 2010;18:153–60. Search in Google Scholar
41. Kuga H, Morisaki T, Nakamura K, Onishi H, Matsuda T, Sueishi K, et al. Construction of a transplantable tissue-engineered artificial peritoneum. Eur Surg Res 2004;36:323–30. Search in Google Scholar
42. Takazawa R, Yamato M, Kageyama Y, Okano T, Kihara K. Mesothelial cell sheets cultured on fibrin gel prevent adhesion formation in an intestinal hernia model. Tissue Eng 2005;11:618–25. Search in Google Scholar
43. Asano T, Takazawa R, Yamato M, Kageyama Y, Kihara K, Okano T. Novel and simple method for isolating autologous mesothelial cells from the tunica vaginalis. BJU Int 2005;96:1409–13. Search in Google Scholar
44. Asano T, Takazawa R, Yamato M, Takagi R, Iimura Y, et al. Transplantation of an autologous mesothelial cell sheet prepared from tunica vaginalis prevents post-operative adhesions in a canine model. Tissue Eng 2006;12:2629–37. Search in Google Scholar
45. Lachaud CC, López-Beas J, Soria B, Hmadcha A. EGF-induced adipose tissue mesothelial cells undergo functional vascular smooth muscle differentiation. Cell Death Dis 2014;5:e1304. Search in Google Scholar
46. Lachaud CC, Soria F, Escacena N, Quesada-Hernandez E, Hmadcha A, et al. Mesothelial cells: a cellular surrogate for tissue engineering of corneal endothelium. Invest Ophthalmol Vis Sci 2014;55:5967–78. Search in Google Scholar
47. Okano T, Yamada N, Sakai H, Sakurai Y. A novel recovery system for cultured cells using plasma-treated polystyrene dishes grafted with poly(N-isopropylacrylamide). J Biomed Mater Res 1993;27:1243–51. Search in Google Scholar
48. Kawanishi K, Yamato M, Sakiyama R, Okano T, Nitta K. Peritoneal cell sheets composed of mesothelial cells and fibroblasts prevent intra-abdominal adhesion formation in a rat model. J Tissue Eng Regen Med 2013. doi:10.1002/term.1860. Search in Google Scholar
49. Inagaki NF, Inagaki FF, Kokudo N, Miyajima A. Cell-based therapy for preventing postoperative adhesion and promoting regeneration after hepatectomy. J Hepatobiliary Pancreat Sci 2015;22:524–30. Search in Google Scholar
50. Haraguchi Y, Shimizu T, Sasagawa T, Sekine H, Sakaguchi K, Kikuchi T, et al. Fabrication of functional three-dimensional tissues by stacking cell sheets in vitro. Nat Protoc 2012;7:850–8. Search in Google Scholar
51. Haraguchi Y, Shimizu T, Yamato M, Kikuchi A, Okano T. Electrical coupling of cardiomyocyte sheets occurs rapidly via functional gap junction formation. Biomaterials 2016;27:4765–74. Search in Google Scholar
52. Körbling M, Freireich EJ. Twenty-five years of peripheral blood stem cell transplantation. Blood 2011;117:6411–16. Search in Google Scholar
53. Burillon C, Huot L, Justin V, Nataf S, Chapuis F, Decullier E, et al. Cultured autologous oral mucosal epithelial cell sheet (CAOMECS) transplantation for the treatment of corneal limbal epithelial stem cell deficiency. Invest Ophthalmol Vis Sci 2012;53:1325–31. Search in Google Scholar
54. Ohki T, Yamato M, Ota M, Takagi R, Murakami D, Kondo M, et al. Prevention of esophageal stricture after endoscopic submucosal dissection using tissue-engineered cell sheets. Gastroenterology 2012;143:582–8. Search in Google Scholar
55. Pronk A, Leguit P, Hoynck van Papendrecht AA, Hagelen E, van Vroonhoven TJ, et al. A cobblestone cell isolated from the human omentum: the mesothelial cell; isolation, identification, and growth characteristics. In Vitro Cell Dev Biol 1993;29A:127–34. Search in Google Scholar
56. Yanez-Mo M, Lara-Pezzi E, Selgas R, Ramirez-Huesca M, Dominguez-Jimenez C, Jimenez-Heffernan JA, et al. Peritoneal dialysis and epithelial-to-mesenchymal transition of mesothelial cells. N Engl J Med 2003;348:403–13. Search in Google Scholar
57. Vargha R, Endemann M, Kratochwill K, Riesenhuber A, Wick N, Krachler AM, et al. Ex vivo reversal of in vivo transdifferentiation in mesothelial cells grown from peritoneal dialysate effluents. Nephrol Dial Transplant 2006;21:2943–7. Search in Google Scholar
58. Yu MA, Shin KS, Kim JH, Kim YI, Chung SS, Park SH, et al. HGF and BMP-7 ameliorate high glucose-induced epithelial-to-mesenchymal transition of peritoneal mesothelium. J Am Soc Nephrol 2009;20:567–81. Search in Google Scholar
59. Loureiro J, Schilte M, Aguilera A, Albar-Vizcaino P, Ramirez-Huesca M, Perez-Lozano ML, et al. BMP-7 blocks mesenchymal conversion of mesothelial cells and prevents peritoneal damage induced by dialysis fluid exposure. Nephrol Dial Transplant 2010;25:1098–108. Search in Google Scholar
60. Sekiguchi Y, Hamada C, Ro Y, Nakamoto H, Inaba M, Shimaoka T, et al. Differentiation of bone marrow-derived cells into regenerated mesothelial cells in peritoneal remodeling using a peritoneal fibrosis mouse model. J Artif Organs 2012;15:272–82. Search in Google Scholar
61. Wang N, Li Q, Zhang L, Lin H, Hu J, Li D, et al. Mesenchymal stem cells attenuate peritoneal injury through secretion of TSG-6. PLoS One 2012;7:e43768. Search in Google Scholar
62. Ueno T, Nakashima A, Doi S, Kawamoto T, Honda K, Yokoyama Y, et al. Mesenchymal stem cells ameliorate experimental peritoneal fibrosis by suppressing inflammation and inhibiting TGF-beta1 signaling. Kidney Int 2013;84:297–307. Search in Google Scholar
63. Kim YD, Jun YJ, Kim J, Kim CK. Effects of human adipose-derived stem cells on the regeneration of damaged visceral pleural mesothelial cells: a morphological study in a rabbit model. Interact Cardiovasc Thorac Surg 2014;19:363–7. Search in Google Scholar
64. Galili U. Discovery of the natural anti-Gal antibody and its past and future relevance to medicine. Xenotransplantation 2013;20:138–47. Search in Google Scholar
65. Salama A, Evanno G, Harb J, Soulillou JP. Potential deleterious role of anti-Neu5Gc antibodies in xenotransplantation. Xenotransplantation 2015;22:85–94. Search in Google Scholar
66. Higginbotham L, Mathews D, Breeden CA, Song M, Farris AB, et al. Pre-transplant antibody screening and anti-CD154 costimulation blockade promote long-term xenograft survival in a pig-to-primate kidney transplant model. Xenotransplantation 2015;22:221–30. Search in Google Scholar
67. Iwase H, Kobayashi T. Current status of pig kidney xenotransplantation. Int J Surg 2015;23:229–33. Search in Google Scholar
68. Lutz AJ, Li P, Estrada JL, Sidner RA, Chihara RK, Downey SM, et al. Double knockout pigs deficient in N-glycolylneuraminic acid and galactose alpha-1,3-galactose reduce the humoral barrier to xenotransplantation. Xenotransplantation 2013;20:27–35. Search in Google Scholar
69. Byrne GW, Du Z, Stalboerger P, Kogelberg H, McGregor CG. Cloning and expression of porcine beta1,4 N-acetylgalactosaminyl transferase encoding a new xenoreactive antigen. Xenotransplantation 2014;21:543–54. Search in Google Scholar
70. Park HM, Park JH, Kim YW, Kim KJ, Jeong HJ, Jang KS, et al. The Xeno-glycomics database (XDB): a relational database of qualitative and quantitative pig glycome repertoire. Bioinformatics 2013;29:2950–2. Search in Google Scholar
71. Estrada JL, Martens G, Li P, Adams A, Newell KA, Ford ML, et al. Evaluation of human and non-human primate antibody binding to pig cells lacking GGTA1/CMAH/beta4GalNT2 genes. Xenotransplantation 2015;22:194–202. Search in Google Scholar
72. Yang L, Guell M, Niu D, George H, Lesha E, Grishin D, et al. Genome-wide inactivation of porcine endogenous retroviruses (PERVs). Science 2015;350:1101–4. Search in Google Scholar
73. Loveland BE, Milland J, Kyriakou P, Thorley BR, Christiansen D, Lanteri MB, et al. Characterization of a CD46 transgenic pig and protection of transgenic kidneys against hyperacute rejection in non-immunosuppressed baboons. Xenotransplantation 2004;11:171–83. Search in Google Scholar
74. Ménoret S, Plat M, Blancho G, Martinat-Botté F, Bernard P, Karam G, et al. Characterization of human CD55 and CD59 transgenic pigs and kidney xenotransplantation in the pig-to-baboon combination. Transplantation 2004;77:1468–71. Search in Google Scholar
75. Elliott RB. Towards xenotransplantation of pig islets in the clinic. Curr Opin Organ Transplant 2011;16:195–200. Search in Google Scholar
76. Orive G, Hernández RM, Rodríguez Gascón A, Calafiore R, Chang TM, de Vos P, et al. History, challenges and perspectives of cell microencapsulation. Trends Biotechnol 2004;22:87–92. Search in Google Scholar
77. Fu RH, Wang YC, Liu SP, Shih TR, Lin HL, Chen YM, et al. Decellularization and recellularization technologies in tissue engineering. Cell Transplant 2014;23:621–30. Search in Google Scholar
78. Hoganson DM, Owens GE, O‘Doherty EM, Bowley CM, Goldman SM, Harilal DO, et al. Preserved extracellular matrix components and retained biological activity in decellularized porcine mesothelium. Biomaterials 2010;31:6934–40. Search in Google Scholar
Mesothelioma – Symptoms and causes
Overview
Malignant mesothelioma (me-zoe-thee-lee-O-muh) is a type of cancer that occurs in the thin layer of tissue that covers the majority of your internal organs (mesothelium).
Mesothelioma is an aggressive and deadly form of cancer. Mesothelioma treatments are available, but for many people with mesothelioma, a cure isn’t possible.
Doctors divide mesothelioma into different types based on what part of the mesothelium is affected. Mesothelioma most often affects the tissue that surrounds the lungs (pleura). This type is called pleural mesothelioma. Other, rarer types of mesothelioma affect tissue in the abdomen (peritoneal mesothelioma), around the heart and around the testicles.
Mesothelioma care at Mayo Clinic
Products & Services
Show more products from Mayo Clinic
Symptoms
Signs and symptoms of mesothelioma vary depending on where the cancer occurs.
Pleural mesothelioma, which affects the tissue that surrounds the lungs, causes signs and symptoms that may include:
- Chest pain
- Painful coughing
- Shortness of breath
- Unusual lumps of tissue under the skin on your chest
- Unexplained weight loss
Peritoneal mesothelioma, which occurs in tissue in the abdomen, causes signs and symptoms that may include:
- Abdominal pain
- Abdominal swelling
- Nausea
- Unexplained weight loss
Other forms of mesothelioma
Signs and symptoms of other types of mesothelioma are unclear, since these forms of the disease are very rare.
Pericardial mesothelioma, which affects tissue that surrounds the heart, can cause signs and symptoms such as breathing difficulty and chest pains.
Mesothelioma of tunica vaginalis, which affects tissue surrounding the testicles, may be first detected as swelling or a mass on a testicle.
When to see a doctor
See your doctor if you have signs and symptoms that worry you. Signs and symptoms of mesothelioma aren’t specific to this disease and, due to the rarity of mesothelioma, are more likely to be related to other conditions. If any persistent signs and symptoms seem unusual or bothersome, ask your doctor to evaluate them. Tell your doctor if you’ve been exposed to asbestos.
Get the latest health information from Mayo Clinic delivered to your inbox.
Subscribe for free and receive an in-depth guide to coping
with cancer, plus helpful advice on how to get a second opinion. You can unsubscribe at any
time.
I would like to learn more about
Subscribe
Learn more about Mayo Clinic’s use of data.
To provide you with the most relevant and helpful information, and understand which
information is beneficial, we may combine your email and website usage information with
other information we have about you. If you are a Mayo Clinic patient, this could
include protected health information. If we combine this information with your protected
health information, we will treat all of that information as protected health
information and will only use or disclose that information as set forth in our notice of
privacy practices. You may opt-out of email communications at any time by clicking on
the unsubscribe link in the e-mail.
Thank you for subscribing
Your in-depth coping with cancer guide will be in your inbox shortly. You will also
receive emails from Mayo Clinic on the latest about cancer news, research, and care.
If you don’t receive our email within 5 minutes, check your SPAM folder, then contact us
at [email protected].
Sorry something went wrong with your subscription
Please, try again in a couple of minutes
Retry
Causes
In general, cancer begins when a series of changes (mutations) happens in a cell’s DNA. The DNA contains the instructions that tell a cell what to do. The mutations tell the cell to grow and multiply out of control. The abnormal cells accumulate and form a tumor.
It isn’t clear what causes the initial genetic mutations that lead to mesothelioma, though researchers have identified factors that may increase the risk. It’s likely that cancers form because of an interaction between many factors, such as inherited conditions, your environment, your health conditions and your lifestyle choices.
Risk factors
Asbestos exposure: The primary risk factor for mesothelioma
Most mesotheliomas are thought to be related to asbestos exposure. Asbestos is a mineral that’s found naturally in the environment. Asbestos fibers are strong and resistant to heat, making them useful in a wide variety of applications, such as in insulation, brakes, shingles, flooring and many other products.
When asbestos is broken up, such as during the mining process or when removing asbestos insulation, dust may be created. If the dust is inhaled or swallowed, the asbestos fibers will settle in the lungs or in the stomach, where they can cause irritation that may lead to mesothelioma. Exactly how this happens isn’t understood. It can take 20 to 60 years or more for mesothelioma to develop after asbestos exposure.
Most people with asbestos exposure never develop mesothelioma. This indicates that other factors may be involved in determining whether someone gets mesothelioma. For instance, you could inherit a predisposition to cancer or some other condition could increase your risk.
Factors that may increase the risk of mesothelioma include:
- Personal history of asbestos exposure. If you’ve been directly exposed to asbestos fibers at work or at home, your risk of mesothelioma is greatly increased.
- Living with someone who works with asbestos. People who are exposed to asbestos may carry the fibers home on their skin and clothing. Exposure to these stray fibers over many years can put others in the home at risk of mesothelioma. People who work with high levels of asbestos can reduce the risk of bringing home asbestos fibers by showering and changing clothes before leaving work.
- A family history of mesothelioma. If your parent, sibling or child has mesothelioma, you may have an increased risk of this disease.
- Radiation therapy to the chest. If you had radiation therapy for cancer in your chest, you might have an increased risk of mesothelioma.
Complications
As pleural mesothelioma spreads in the chest, it puts pressure on the structures in that area. This can cause complications, such as:
- Difficulty breathing
- Chest pain
- Difficulty swallowing
- Pain caused by pressure on the nerves and spinal cord
- Accumulation of fluid in the chest (pleural effusion), which can compress the lung nearby and make breathing difficult
Prevention
Reducing your exposure to asbestos may lower your risk of mesothelioma.
Find out whether you work with asbestos
Most people with mesothelioma were exposed to the asbestos fibers at work. Workers who may encounter asbestos fibers include:
- Asbestos miners
- Electricians
- Plumbers
- Pipefitters
- Insulators
- Shipyard workers
- Demolition workers
- Brake mechanics
- Selected military personnel
- Home remodelers
Ask your employer whether you have a risk of asbestos exposure on the job.
Follow your employer’s safety regulations
Follow all safety precautions in your workplace, such as wearing protective equipment. You may also be required to shower and change out of your work clothes before taking a lunch break or going home. Talk to your doctor about other precautions you can take to protect yourself from asbestos exposure.
Be safe around asbestos in your home
Older homes and buildings may contain asbestos. In many cases, it’s more dangerous to remove the asbestos than it is to leave it intact. Breaking up asbestos may cause fibers to become airborne, where they can be inhaled. Consult experts trained to detect asbestos in your home. These experts may test the air in your home to determine whether the asbestos is a risk to your health. Don’t attempt to remove asbestos from your home — hire a qualified expert.
Oct. 20, 2020
Types of Mesothelioma
Types of Mesothelioma include pleural mesothelioma, peritoneal mesothelioma and pericardial mesothelioma. Mesothelioma cancer affects the mesothelial tissue lining of the body’s three largest cavities; the pleura (lung cavity), the peritoneum (abdominal cavity) and the pericardium (heart sac). A type of malignant mesothelioma is based on the section of the mesothelium that it affects.
Pleural Mesothelioma
Pleural mesothelioma is the most common type of malignant mesothelioma (accounting for an approximate 75% of all documented cases of the disease) and affects the section of the mesothelium called the pleura. Although the most common type of malignant mesothelioma, the disease is still somewhat of a rarity. As a result, pleural mesothelioma is often confused with other types of diseases, such as lung cancer and viral pneumonia. Lung cancer can be caused by asbestos (asbestos lung cancer), though it differs from pleural mesothelioma in that it is a malignancy of the lung tissue itself, as opposed to pleural mesothelioma which is a malignancy of the tissue casing of the lungs. Viral pneumonia shares certain symptomatic similarities with pleural mesothelioma and is often misdiagnosed as such.
The most common presenting symptom of pleural malignant mesothelioma is chronic chest pain. A buildup of fluid inside the pleural space can cause severe and chronic chest pains; this is called pleural effusion. Steps can be taken to drain the fluid and relieve the pain (with the possibility of recurrence) or surgery can be performed to close the pleural space (with virtually no possibility of recurrence). Some of the other notable symptoms associated with pleural mesothelioma include:
- Shortness of breath
- Chronic coughing
- Weight loss
- Fever
more info about Pleural Mesothelioma
Pericardial Mesothelioma
Pericardial mesothelioma is much less common than malignant mesothelioma of the pleura or peritoneum. In fact there are only about 150 cases ever reported in the medical literature. It affects the section of the mesothelium called the pericardium (the mesothelial lining of the heart). People in the fourth to seventh decades of life are most likely to have this cancer, and there is a 2:1 male to female ratio. Currently, surgical excision (removal) of the pericardium is the treatment for pericardial mesothelioma, primarily to lessen symptoms of constriction around the heart.
Symptoms that are associated with pericardial mesothelioma include:
- Chest pain
- Fluid buildup around the heart
- A mass in the space between the lungs
- Abnormal or difficult breathing (dyspnea)
- Chronic coughing
- Irregular heartbeat (palpitations)
more info about Pericardial Mesothelioma
Mesothelioma of the Tunica Vaginalis Testis
Mesothelioma of the tunica vaginalis testis is the least common type of malignant mesothelioma (amounting to less than 100 of all documented cases of the disease) and affects the section of the mesothelium called the tunica vaginalis testis (the mesothelial lining around the testes). Most patients are in their 50s or older, but about ten percent of the patients are younger than 25 years. Patients generally present with a hydrocele (an accumulation of serous fluid in a sac-like cavity (as the scrotum)) or hernia. Treatment is usually a high inguinal orchiectomy (surgical excision of the entire affected testis through an incision in the lower abdomen – called also orchidectomy). Prognosis is somewhat better than for pleural mesothelioma.
Symptoms that are associated with this cancer include:
- Hydrocele (a fluid filled sac attached to a testicle)
- Suspected hernia
more info about Mesothelioma Tunica
Peritoneal Mesothelioma
Peritoneal mesothelioma is the second most common type of malignant mesothelioma (accounting for an approximate 10% to 20% of all documented cases of the disease) and affects the section of the mesothelium called the peritoneum (the mesothelial lining of the abdomen). Peritoneal mesothelioma is most often caused by the ingestion of carcinogenic asbestos fibers. Inhaled asbestos fibers can become lodged in mucous lining the mouth and esophagus. Once swallowed, it travels through the digestive system where it can potentially become lodged and develop into a tumor.
Some of the notable symptoms that are associated with peritoneal mesothelioma include:
- Swelling
- Abdominal pain (resulting from fluid buildup in peritoneal space)
- Weight loss
- Abdominal mass
- Lowered red blood cell count (anemia)
- Fever
- Bowel obstruction
- Blood clotting problems
more info about Peritoneal Mesothelioma
Top of Page
JDB | Free Full-Text | Development of the Serosal Mesothelium
1. Mesothelia: Broad Definition
Mesothelia are simple squamous epithelia that line coelomic cavities and organs and form the mesenteries. In mammals, separation of the common embryonic coelom gives rise to pericardial, pleural and peritoneal cavities (discussed in detail below). Mesothelial cells lining the cavities/body walls in these subdivisions are referred to as parietal mesothelia while visceral mesothelia cover the organs. Visceral mesothelium covering the heart is referred to as the epicardium while pleural mesothelium covers lungs. Serosal mesothelium, which is the focus of this review, covers the organs of the alimentary canal within the abdominal cavity/coelom (Figure 1).
Figure 1.
Mesothelia. Parietal mesothelia (thick black lines) cover the body wall and pericardial sac. Visceral mesothelia cover the organs and mesenteries and form a continuous lining with the parietal mesothelia. LI, large intestine; P, pancreas; S, spleen; SI, small intestine; St, stomach.
Figure 1.
Mesothelia. Parietal mesothelia (thick black lines) cover the body wall and pericardial sac. Visceral mesothelia cover the organs and mesenteries and form a continuous lining with the parietal mesothelia. LI, large intestine; P, pancreas; S, spleen; SI, small intestine; St, stomach.
Below, we describe the histology of adult mesothelium and give a brief overview of heart and intestinal structure. This is followed by a discussion of similarities and differences in basic developmental programs of the two organs as a guidepost for understanding the roles of mesothelia in organ development and function. We will then provide an overview of proepicardium/epicardium differentiation to serve as the departure point for the current discussion of mesothelial differentiation in the gut tube and its associated glands.
2. Adult Mesothelia: Cell Biology, Histology, and Function
Despite the multiple names, mesothelium has a relatively consistent structure in each of the coelomic cavities [1,2] (Figure 2). The structure of mesothelium is that of a simple squamous epithelium; a single layer of thin plate-like cells [3]. Histologically, there are three broad characteristics that define this epithelium: apical/basolateral polarity, robust cell/cell adhesion, and production of a basement membrane. Mesothelia are polarized with their apical surfaces toward the coelomic space. Apical surface modifications may include microvilli and cilia [4,5,6]. Tight junctions are located on the lateral aspect of the cell and evidenced by the localization of Bves and ZO-1 [7,8]. They maintain apical/basal polarity and create a diffusion barrier between the coelomic space and the submesothelial connective tissue of the organ or body wall. E-cadherin confers further cell-cell adhesion and cytokeratins (mostly subtypes 8, 18, and 19) provide structural support [9,10] (Figure 2B). A basement membrane is produced by and underlies the mesothelium separating it from the sub-mesothelial connective tissue space (Figure 2C).
Figure 2.
Mesothelial cell structure. (a) Transverse section through a chick embryonic day 8 small intestine. The mesothelium has attained a mature configuration by this developmental stage. (b–e) Magnified view of boxed area shown in A. (b) Mesothelial cells (arrows) are cytokeratin (CK, red) positive. (c) A laminin (lam, green) positive basement membrane underlies the mesothelium. (d) Mesothelial nuclei are flat in shape (arrows). (e) Merge of B–D.
Figure 2.
Mesothelial cell structure. (a) Transverse section through a chick embryonic day 8 small intestine. The mesothelium has attained a mature configuration by this developmental stage. (b–e) Magnified view of boxed area shown in A. (b) Mesothelial cells (arrows) are cytokeratin (CK, red) positive. (c) A laminin (lam, green) positive basement membrane underlies the mesothelium. (d) Mesothelial nuclei are flat in shape (arrows). (e) Merge of B–D.
The most conspicuous function of mesothelia in the adult is the production of a non-adhesive surface allowing relatively frictionless movement of the viscera within the individual coelomic cavity. This is accomplished by secretion of an apical glycosaminoglycan layer and production of a small amount of circulating coelomic fluid to provide lubrication [11,12]. On the surface of the diaphragm, there are gaps in the mesothelial monolayer called stomata that can directly connect with submesothelial lymphatic vessels [6]. Not only is ionic and protein composition of coelomic cavity fluid regulated by mesothelium, but passage of inflammatory cells is also restricted [13,14,15]. Additionally, mesothelium is crucial to the injury response and is thought to both prevent and promote scarring depending on the injury and to promote neovascularization [16,17,18,19]. These and many other studies have provided growing evidence for the dynamic role of mesothelium in both normal and abnormal physiologic states in the adult [20].
3. Heart Histology
The human heart is a muscular pump with complex valvular and neuronal components and an elaborate conduction system. Still, the histology of the heart wall is relatively simple. From the lumen outward, the first cell type seen in any part of the vertebrate heart is the endocardial endothelial cell (Figure 3). This cell rests upon a scant connective tissue layer that merges with the myocardium [5]. The myocardium is populated by cardiac myocytes and contains abundant fibroblasts, which actually outnumber the cardiomyocytes, as well as smooth muscle and endothelial cells of arteries, veins, and capillaries and migratory cells of the immune system. On its surface, the heart is covered by the epicardium composed of an outermost mesothelium and underlying connective tissue space inhabited by fibroblasts, adipose cells and matrix. The major coronary vessels run within this connective tissue space with smaller branches diving into the substance of the myocardium [21]. Each myocyte is invested with a capillary bed. Proximally, the coronary vessels connect with the aorta. There is continuity between the mesothelia of the epicardium and pericardium as well as the mesocardium, the mesentery-like structure that connects the heart to the pericardial sac and dorsal body wall [22]. All the cells composing the heart, with the exception of neural crest derivatives and migratory cells of the immune system are generated from splanchnic mesoderm. There is no endodermal component to the heart. Murine and avian cardiac structure is similar to the human configuration though notably in the mouse the coronary arteries are embedded within the myocardium near the subepicardial space while the veins reside in the epicardium [23]. In the avian heart, the arteries are localized to the epicardial connective tissue while the veins are embedded within the myocardium [24,25]. In summary, the muscular and connective tissue layer of the heart is bounded by a luminal (endocardium) and surface (mesothelium) epithelium with an arterial supply that is associated closely with the surface mesothelium (Figure 3A).
Figure 3.
Structure of the human heart and intestine. (a) The lumen of the heart is lined by endocardium (green line), an endothelial cell type. The myocardium composed of myocytes and connective tissue cells forms the majority of the wall (bracket). Mesothelium lines the surface of the heart. The coronary arteries run in the connective tissue layer (light gray) subjacent to the mesothelium. Branches of the coronary arteries penetrate the myocardium. (b) The lumen of the intestine is lined by mucosal epithelium (M, yellow). There are multiple layers of connective tissue and muscle (bracket) underlying the mucosal epithelium. The first connective tissue layer is the lamina propria (LP, gray). The submucosa (S, tan), another connective tissue layer, encircles the lamina propria. The muscularis externus (ME) is composed of two layers of visceral smooth muscle (red). Serosal mesothelium (SM) lines the surface of the intestine. The major blood vessels of the intestine course through the submesothelial connective tissue (light gray) and branches of the vessels penetrate into the intestinal wall.
Figure 3.
Structure of the human heart and intestine. (a) The lumen of the heart is lined by endocardium (green line), an endothelial cell type. The myocardium composed of myocytes and connective tissue cells forms the majority of the wall (bracket). Mesothelium lines the surface of the heart. The coronary arteries run in the connective tissue layer (light gray) subjacent to the mesothelium. Branches of the coronary arteries penetrate the myocardium. (b) The lumen of the intestine is lined by mucosal epithelium (M, yellow). There are multiple layers of connective tissue and muscle (bracket) underlying the mucosal epithelium. The first connective tissue layer is the lamina propria (LP, gray). The submucosa (S, tan), another connective tissue layer, encircles the lamina propria. The muscularis externus (ME) is composed of two layers of visceral smooth muscle (red). Serosal mesothelium (SM) lines the surface of the intestine. The major blood vessels of the intestine course through the submesothelial connective tissue (light gray) and branches of the vessels penetrate into the intestinal wall.
4. Gut Tube Histology
Structure of the adult vertebrate gastrointestinal (GI) tract with all its associated glands, is highly variable. Still, these differences are actually variations of a basic or conserved structure. Modifications of four generalizable layers account for the variations observed in the stomach and intestines. Understanding the positions and relative contributions of these basic layers is important in understanding gut tube structure and function. The four conserved layers of the gut from the lumen outward are: (1) mucosa, (2) submucosa, (3) muscularis externa and (4) serosa [5] (Figure 3B).
These layers vary in composition and thickness along the length of the gut tube but are always present within organs of the alimentary canal housed within the abdominal cavity. The mucosa consists of an epithelial layer bordering the lumen and a subjacent connective tissue layer called the lamina propria. Mucosal epithelium along the entire gut tube and its associated glands is derived from endoderm (further discussion on this topic is given below). This is in contrast to the heart where no endodermal component is found. The major function of the mucosa is secretion and absorption of luminal substances. The submucosa is also rich in connective tissue cells and fibers. Glands, whose epithelial secretory and ductal components are continuous with the mucosal epithelium, may reside within the lamina propria and/or submucosa. Along with the lamina propria, the submucosa provides support for the distention and contraction of the GI wall. Smooth muscle of the muscularis externa responsible for moving luminal content along the alimentary canal is present between the submucosa and the connective tissue layer of the serosa. The outermost layer of the alimentary canal is the serosa with its connective tissue and mesothelium. As stated above, the major function of the serosa in the adult is provision of a smooth, lubricated surface upon which alimentary organs can move within the abdominal cavity.
During development, the major blood vessels to the gut, the celiac and superior and inferior mesenteric arteries, form as unpaired branches of the aorta and course to the gut within a dorsal mesentery. While the celiac and superior mesenteric arteries remain intraperitoneal, the inferior mesenteric artery and much of the territory it supplies becomes secondarily retroperitoneal as development proceeds. In the adult, the large branches of these arteries reside near the surface of the organs of the gastrointestinal tract in the sub-mesothelial space, just like the major vessels of the heart [22]. As these major vessels traverse the surface of the gut, they send branches down into the substance of the muscularis externa, submucosa, and lamina propria ending in capillary networks serving these regions (Figure 3B). While capillaries are intimately associated with the epithelial component of the mucosa, they do not penetrate the epithelium under normal conditions. Thus, similar to the heart, the stomach and intestine have muscular and connective tissue layers bounded by a luminal (mucosal) and surface (mesothelial) epithelium. Vessels of both the cardiac and alimentary systems travel in the submesothelial space and dive into the substance of the organ giving rise to a capillary plexus that is essential for organ function. However, unlike the heart, the luminal epithelium of the GI tract is derived from endoderm and endothelial cells are not found within this layer (Figure 3). Even from this brief description, it is clear that there are remarkable structural similarities between the heart and GI tract especially between mesothelial components and the associated vascular systems.
5. Coelom Formation, Coelomic Organs, and Mesothelia
Some of the structural variation and similarity can be explained by basic developmental principles of coelom formation and organogenesis in vertebrates. Understanding these principles also establishes the groundwork for a discussion of mesothelial development.
True coelomates contain a fluid filled cavity lined entirely by mesoderm. In vertebrates, this in generated through splitting the lateral plate mesoderm into two subdivisions separated by an intervening space (the coelom) very early in development. The dorsally located lateral plate mesoderm that associates with the ectoderm is called the somatic mesoderm and will form the body wall. The more ventral lateral plate mesoderm (splanchnic mesoderm) which associates with endoderm is destined to build organs of the coelomic cavity (Figure 4A). Splanchnic mesoderm and endoderm are collectively termed splanchnopleure while somatic mesoderm together with ectoderm is referred to as somatopleure. It is interesting to note that a vascular plexus forms between the endodermal and splanchnic mesodermal layers of the embryo [20,26,27] (Figure 4A, green). Concurrent with these events, the embryo begins to take on a cylindrical shape with lateral folding (Figure 4A, arrows). These morphogenetic movements reposition the three germ layers such that a “tube within a tube” is formed (Figure 4B–C). It is important to consider that there is variation in the positioning of endoderm relative to splanchnic mesoderm along the anteroposterior axis of the developing coelom. In the anterior region of the embryo, where the heart will form, endoderm is positioned dorsal to the forming coelomic cavity (Figure 4B). This explains the position of the esophagus and trachea in the dorsal body wall of the adult vertebrate. The dorsal position of the endoderm in the anterior region of the embryo has important implications for heart development. Specifically, the vascular plexus situated between the endoderm and splanchnic mesoderm throughout the rest of the embryo is engaged to contribute to the luminal endocardial layer of the heart tube [28] (Figure 4B). Thus, even while blood courses through the heart lumen, the heart primordium itself is avascular throughout the initial stages of organogenesis. More posteriorly, beginning in the region of the forming septum transversum, endoderm is more ventrally deposed and is enveloped by splanchnic mesoderm (Figure 4C). Given the continuing juxtaposition of endoderm and splanchnic mesoderm in the forming gut, the intervening vascular plexus is retained in this location throughout development.
It is interesting to note that the entire coelomic space is always bounded by mesoderm; indeed, this is the landmark of a true coelomate. Great variation in the structures derived from somatopleure and splanchnopleure is observed in true coelomates and subdivision of the original coelom is highly variable. Still, the basic spatial relationship of somatopleure, coelom, and splanchnopleure is maintained throughout the diversity of true coelomates and the juxtaposition of endoderm and mesoderm is a major step in evolution allowing the embryo to diversify by regionalizing function.
While the coelomic space is bounded by mesoderm, this does not mean that a mesothelium is present throughout development. In fact, the vertebrate coelom and its organs, specifically the heart and elements of the digestive system, are initially devoid of mesothelia [26,27,29,30]. While work in this area has been principally focused on avian and mammalian embryos, it is likely that other vertebrate classes develop similarly.
Thus, at the end of lateral folding, the heart is composed of two layers—endocardium and splanchnic mesoderm. The gut tube is also composed of two layers—endoderm and splanchnic mesoderm—but contains an endothelial plexus between the two layers. Neither the heart or gut tube is bordered on the coelomic surface by a mesothelium.
Figure 4.
Heart and gut tube formation. (a) Schematic of a transverse section through the posterior region of a Hh24 chick embryo demonstrating the relationship of the splanchnic mesoderm and endoderm prior to lateral folding. The lateral plate mesoderm is divided to form the somatic (So) and splanchnic mesoderm (Sp) with an intervening coelomic cavity (CC). An endothelial plexus (EP, green) resides between the splanchnic mesoderm and endoderm (E, yellow). Lateral folding (arrows) will unite the somatopleure and splanchnopleure ventrally. (b) In the anterior region of the Hh24 embryo, lateral folding has already been completed. The endoderm has been excluded from the heart tube (HT) and cells of the endothelial plexus have formed the endocardial (EC) lining of the heart tube lumen. Dorsal to the heart tube, the endoderm of the gut tube remains invested by the endothelial plexus and splanchnic mesoderm. (c) Posterior to the heart tube in a Hh28 chick embryo, the gut tube is the only structure within the coelomic cavity and includes endoderm, the endothelial plexus, and splanchnic mesoderm. DA, dorsal aorta; I, intermediate mesoderm; N, notochord; NT, neural tube; S, somite.
Figure 4.
Heart and gut tube formation. (a) Schematic of a transverse section through the posterior region of a Hh24 chick embryo demonstrating the relationship of the splanchnic mesoderm and endoderm prior to lateral folding. The lateral plate mesoderm is divided to form the somatic (So) and splanchnic mesoderm (Sp) with an intervening coelomic cavity (CC). An endothelial plexus (EP, green) resides between the splanchnic mesoderm and endoderm (E, yellow). Lateral folding (arrows) will unite the somatopleure and splanchnopleure ventrally. (b) In the anterior region of the Hh24 embryo, lateral folding has already been completed. The endoderm has been excluded from the heart tube (HT) and cells of the endothelial plexus have formed the endocardial (EC) lining of the heart tube lumen. Dorsal to the heart tube, the endoderm of the gut tube remains invested by the endothelial plexus and splanchnic mesoderm. (c) Posterior to the heart tube in a Hh28 chick embryo, the gut tube is the only structure within the coelomic cavity and includes endoderm, the endothelial plexus, and splanchnic mesoderm. DA, dorsal aorta; I, intermediate mesoderm; N, notochord; NT, neural tube; S, somite.
6. Development of the Epicardium
A review of the literature quickly reveals that relatively little is known about the origin of mesothelia and its “arrival” on organs with the notable exception of cardiac development. We provide a brief overview of proepicardium/epicardium development to inform the current discussion of mesothelial differentiation in the gut tube and its associated glands. Excellent and extensive reviews focused on epicardial development are available to the reader in this edition.
As stated above, as the heart tube begins its characteristic looping, it is devoid of mesothelium. The outer cell layer of the developing heart is the myocardium, which is a polarized epithelium with its basal surface facing the endocardial space and its apical side facing the coelom [26,30]. At ED8.5 in mouse and HH stage 14 in chick, a simple squamous epithelial outgrowth from the region of the sinus venosus courses through the pericardial coelom [29,31] (Figure 5A). This structure is termed the proepicardium or PE and is not simply an epithelium but also houses cells within its connective tissue space. These cells may be the product of epithelial to mesenchymal transition (EMT) from the migrating epithelium [32] or simply cells that travel with this migratory structure. In the avian embryo, the PE extends villi in close approximation to proteoglycan-rich connective tissue fibrils to reach the heart [31] (Figure 5B, arrowhead). Apical/basal polarity of the epithelial PE, in the chicken embryo at least, is observed as soon as the PE can be identified and is maintained throughout the life of a healthy bird. Nahirney et al. have shown that once the PE reaches the myocardium, the clusters open (Figure 5C, arrowhead) such that two sheets of simple squamous epithelium travel on the heart wall (Figure 5C, arrows) and eventually envelop it [31] (Figure 5D). In this way, the polarity of the PE/epicardium is maintained with the apical surface facing the coelomic cavity. Retention of epithelial polarity is essential in positioning the mesothelium for its functions in homeostasis and production of mesenchyme in organogenesis.
Figure 5.
Proepicardium (PE) development. (a) The PE (blue) forms external to the heart tube as an outgrowth from the region of the sinus venosus (SV) and liver bud (LB). (b) The PE epithelium is polarized with apical toward the pericardial space. The PE crosses the pericardial cavity to contact (arrowhead) the myocardium (M). (c) At points of contact with the myocardium, the PE epithelial sheet breaks open (arrowheads) to preserve apical-basal polarity and migrate over the heart tube (arrows). (d) During migration and after a continuous epicardium (Ep) is formed, cells leave the epicardium (arrowheads) via epithelial to mesenchymal transition (EMT) to migrate into the myocardium and generate fibroblasts (F) and the coronary blood vessels (BV). EC, endocardium; G, gut tube; H, heart tube.
Figure 5.
Proepicardium (PE) development. (a) The PE (blue) forms external to the heart tube as an outgrowth from the region of the sinus venosus (SV) and liver bud (LB). (b) The PE epithelium is polarized with apical toward the pericardial space. The PE crosses the pericardial cavity to contact (arrowhead) the myocardium (M). (c) At points of contact with the myocardium, the PE epithelial sheet breaks open (arrowheads) to preserve apical-basal polarity and migrate over the heart tube (arrows). (d) During migration and after a continuous epicardium (Ep) is formed, cells leave the epicardium (arrowheads) via epithelial to mesenchymal transition (EMT) to migrate into the myocardium and generate fibroblasts (F) and the coronary blood vessels (BV). EC, endocardium; G, gut tube; H, heart tube.
It is of interest to note that when the PE can first be identified, its epithelial component expresses gene products characteristic of a mesothelium including Tbx18, Wt1, and Tcf21 [33,34,35]. Thus, before these cells come in contact with their target organ the heart, they appear to have already differentiated, or at least partially so, into a mesothelium.As the epicardium advances over the developing myocardium, the process of EMT begins. EMT is well underway before the epicardium fully covers the heart and several laboratories have demonstrated the propensity of the epicardium to form mesenchymal cells [8,33,36,37]. The distribution of epicardially-derived mesenchyme is not even throughout the heart with the bulk observed at the atrioventricular junction where major vessels will develop at subsequent stages. Other myocardial regions are not so heavily populated by EMT-derived cells [8]. The identity of specific EMT-derived progeny is firmly established while that of others is currently in debate. Direct labeling of the PE with vital dyes and retroviral lineage tracers in avian embryos has demonstrated that precursors of the epicardium, vascular endothelial and smooth muscle cells as well as pericytes and fibroblasts are housed within the PE [32,38,39]. Conversely, ablation of the PE results in loss of the coronary vasculature [40]. Previous studies tracing the Wt1 and Tbx18 proepicardial lineages suggested that endothelial cells may not be the descendants of the epithelial PE but potentially arise from its mesenchymal component [34,35]. A more recent study determined that endothelial cells are indeed derived from specific compartments of the PE that express Scleraxis and Semaphorin3D [41]. Taken together, studies on the PE/epicardium suggest that these simple squamous epithelial structures give rise to the vast majority of cells comprising the coronary vessels and cardiac fibroblasts. Another important aspect to generation of the coronary arterial system is the growth and maturation of the proximal portion of these vessels. Manner demonstrated that the proximal ends of coronary vessels move within the epicardial space toward the aorta [42]. These vessels penetrate the tunica media of the aorta and through apoptotic events around the future coronary orifice establish continuity with the aorta and systemic circulation [43]. Prior to the establishment of the final coronary arterial pathway, intermittent passage of blood is observed through transient patency of coronary sprouts with the aorta [44]. This mechanism of vasculogenesis in developing organs has only been reported for the heart.
To summate, the heart, which is housed within the pericardial cavity is initially devoid of a mesothelium and blood vessels. The PE migrates to and over the heart tube with a concurrent EMT-driven production of freely migratory cells. These cells differentiate into vascular endothelium and smooth muscle as well as cardiac fibroblasts and possibly cardiac myocytes. Thus, the PE is the major source of cells for the developing coronary system as well as other fibroblastic and parenchymal cell types in the entire organ.
New and interesting studies will be published on the regulation of PE/epicardial control of cardiac development but it appears that the major roadmap of development is understood. An intriguing question arises, “Is this developmental process conserved in the formation and development of other coelomic organs?” In contemplating this question, we became particularly interested in the GI tract due to the similar structural arrangement of the intestinal and cardiac vasculature, as described above.
7. Development of the Serosal Mesothelium
As noted earlier, the alimentary canal arises from two embryonic structures: endoderm and splanchnic mesoderm. Later, neural crest cells invade and contribute to elements of the enteric plexus [45]. With lateral folding, a complete tube is suspended from the dorsal body wall by a dorsal mesentery not unlike the early heart. The epithelial endoderm is maintained as the GI mucosal epithelium but also buds into the surrounding mesoderm in highly regulated processes that produce the epithelial components of glands such as the liver and pancreas. The epithelial connections between the intestinal mucosa and the glandular epithelium of these exocrine organs via continuous ductal systems are maintained throughout life. Concurrent with this epithelial morphogenesis, cells of the splanchnic mesoderm undergo complex remodeling to produce connective tissue, visceral smooth muscle, and vascular components of the gastrointestinal wall and glands. Excellent reviews examine the signaling and genetic control of this process [46,47]. For purposes of the current discussion, it is important to note that stromal elements of the lamina propria and submucosa along with those same constituents in associated glands are thought to be progeny of the splanchnic mesoderm. Further visceral smooth muscle of the muscularis externa is also descendent from splanchnic mesoderm. As noted above, neural crest cells migrate into the GI tract to produce neurons and glia of the enteric plexus [45]. In 1980 Meier answered a fundamental question, “When is the mesothelium first seen in gut development?” He demonstrated that splanchnic mesoderm was initially devoid of a histologically identifiable mesothelium. We later demonstrated that the gut tube did not initially express molecular markers (Wt1, cytokeratin) of the mesothelial phenotype [27,48]. It was interesting to note that in the mouse, expression of Wt1, a widely used marker for mesothelial cells, was first seen at the root of the dorsal mesentery of the intestine and subsequently spread ventrally over the gut tube. We postulated that a wave of migratory cells, like those of the PE swept down upon the developing gut tube though it should be noted this has never been tested.In a recently published communication, we detailed the formation of mesothelium on the gut wall in the avian embryo [26]. Using the quail embryo as a developmental model, we confirmed Meier’s finding that splanchnic mesoderm is devoid of mesothelium. With the split of lateral mesoderm to form the coelom, the gut organ primordium is composed solely of endoderm, splanchnic mesoderm and the aforementioned vascular plexus which is situated between their basement membranes (Figure 6A–B). Our present data suggest that cells of the splanchnic mesoderm lose their epithelial nature and move into the space between juxtaposed basement membranes forming a primary gut mesenchyme (Figure 6C). This mesenchyme will give rise to cellular components of the lamina propria, submucosa, and muscularis externa. All these cells are non-epithelial and are grouped as “connective tissue/stromal/smooth muscle” cells. The inner endodermally-associated basement membrane is definitively retained throughout development. The outer mesodermally-associated basement membrane, while always present, is temporally fragmented during the course of organogenesis (Figure 6C). We emphasize the positioning and constancy of these two basement membranes as they represent important landmarks for an understanding of intestinal and, specifically, mesothelial development. These are the only two continuous basement membranes in the entirety of the developing and adult gut tube. The inner basement membrane is associated with the mucosal epithelium and with its precursor embryonic endoderm. The outer basement membrane is originally associated with splanchnic mesoderm but where is its position in the adult and where is the mesothelium in relationship to this important landmark? The outer epithelium is highly dynamic during this initial timeframe. The epithelium itself changes shape and thickness with many cells moving from the surface into the mesenchymal space between the two basement membranes. At these times, this outer epithelium ranges from columnar to pseudostratified to stratified but is clearly not simple squamous in appearance. Only at embryonic day 6 of chick or quail development is definitive mesothelium seen on the forming gut tube [26,27]. After this time and extending throughout adulthood, the simple squamous mesothelium is the only cell type seen external to the outer basement membrane. Once the mesothelium has been established, we have shown in the mouse that EMT from this epithelium ensues [48]. With time, a connective tissue space is established subjacent to the basement membrane with an embedded vasculature. This is the same arrangement observed in the heart with its epicardium. Thus, at the end of development, the surface of the heart and GI tract have the same arrangement of mesothelium and underlying connective tissue with a vascular supply. But, is there a “gut PE”?
Figure 6.
Intestinal mesothelial development. (a) Schematic depicting an early embryo yet to undergo lateral folding. (b) The splanchnopleure of the intestinal anlage (boxed area in A) is composed of splanchnic mesoderm (Sp) and endoderm (E) with an intervening endothelial plexus (EP). The mesothelial progenitors (blue) are distributed primarily near the surface of the stratified splanchnic mesoderm while fibroblast and visceral smooth muscle progenitors are deep (red). (c) Cells of the splanchnic mesoderm undergo EMT and invade the mesenchymal space joining the endothelial plexus to form the mesenchymal layer (ML). The mesodermal basement membrane breaks down through this process (broken black line). (d) A simple squamous mesothelium (blue) is present on the surface by day 6 of chick development and E11.5 of mouse development. Mesothelial cells undergo EMT to give rise to vascular smooth muscle. CC, coelomic cavity; DA, dorsal aorta; E, endoderm; I, intermediate mesoderm; N, notochord; NT, neural tube; S, somite.
Figure 6.
Intestinal mesothelial development. (a) Schematic depicting an early embryo yet to undergo lateral folding. (b) The splanchnopleure of the intestinal anlage (boxed area in A) is composed of splanchnic mesoderm (Sp) and endoderm (E) with an intervening endothelial plexus (EP). The mesothelial progenitors (blue) are distributed primarily near the surface of the stratified splanchnic mesoderm while fibroblast and visceral smooth muscle progenitors are deep (red). (c) Cells of the splanchnic mesoderm undergo EMT and invade the mesenchymal space joining the endothelial plexus to form the mesenchymal layer (ML). The mesodermal basement membrane breaks down through this process (broken black line). (d) A simple squamous mesothelium (blue) is present on the surface by day 6 of chick development and E11.5 of mouse development. Mesothelial cells undergo EMT to give rise to vascular smooth muscle. CC, coelomic cavity; DA, dorsal aorta; E, endoderm; I, intermediate mesoderm; N, notochord; NT, neural tube; S, somite.
To determine whether gut mesothelium arises from an exogenous source like that seen in the heart, we employed a chick/quail chimeric system where segments of quail splanchnopleure (endoderm plus splanchnic mesoderm) were transplanted into a host chick coelomic cavity. Interestingly, mesothelium was generated from the graft tissue and not the host, meaning that mesothelial progenitors were broadly positioned and intrinsic to the organ primordium, and that a “gut PE” does not account for these cells [27]. This was substantiated by DNA electroporation and long term retroviral experiments labeling surface cells of splanchnic mesoderm. Taken together, our recently published data suggest that the mesothelium of the avian gut tube is derived by a mechanism completely different from that seen in the heart.
10. Lineages Generated From Epicardial and Serosal Mesothelia
Numerous studies have analyzed the cell types generated by the PE/epicardium. In studies of heart organogenesis, these cells generated through EMT include vascular smooth muscle, fibroblasts, myofibroblasts, endothelium, and possibly cardiac myocytes, as well as the epicardium itself (see above and other articles in this issue). Lineage tracing studies of proepicardial cells have been conducted using direct retroviral labeling as well as genetic techniques. Results vary with these two methodologies as one labels a “location” while the other labels an “expressing cell”. Results from the two labeling strategies demonstrate the presence of both endothelial and vascular smooth muscle progenitors within the PE [32,38,39,40,41,48,53,54] although positioning of these progenitors within the PE is still a matter of question. In reflecting on the differentiation of mesothelial cells in the developing gut tube, the aforementioned types of experiment are difficult to interpret in the context of gut organogenesis. First, no one has proven that gut mesothelium is the only population to express the currently available mesothelial genetic markers (such as Wt1, Tbx18, or Gata4). In Wilm et al [48], we demonstrated that the Wt1 genetic lineage gives rise to the majority of vascular smooth muscle of the intestines. However, our recent data suggest that Wt1, an often used marker, is expressed in splanchnic mesoderm prior to generation of a definitive mesothelium [27]. This is also observed in a recent study genetically tracing a mesothelin-positive lineage throughout the coelomic cavity in the mouse. This particular gene, though associated with differentiated mesothelium, was demonstrated to also label a progenitor population, termed Fibroblast and Smooth Muscle Cell (FSMC) precursors, that embryonically gives rise not only to mesothelium but also the vast majority of visceral smooth muscle of the intestines and bladder [55]. Going forward, it will be important to carefully characterize the expression of “mesothelial-specific” genes before standard Cre tracing systems can be used to study mesothelially-derived cell lineages in the gut tube, lungs, and associated glands. This would include any work from our own laboratory. Retroviral, vital dye, or DNA electroporation-based labeling of cells at or near the surface of intestinal splanchnic mesoderm marks definitive mesothelium as well as vascular smooth muscle and stromal cells within the connective tissue if the embryo is allowed to grow [27]. At the time of labeling in these experiments, the splanchnic mesoderm is devoid of a definitive mesothelial cell layer and is characterized by stratified and pseudostratified epithelium. Thus, while these experiments definitively demonstrate that mesothelial progenitors reside within the gut primordium identifying labeled cells as strict descendants of the gut mesothelium is problematic.Fundamental differences in mesothelial development in the heart and gut account for the difficulty in interpretation of mesothelial descendants in the developing GI tract. First, the mesothelial precursor of the heart, the PE, has characteristics of a mesothelium before it arrives at its target organ. Thus, expression analysis of genes to be used for lineage-tracing can be more easily conducted. Still, even now, controversy in data interpretation has been experienced. Conversely, precursors of gut mesothelium arise broadly within the developing organ and are heterogeneously dispersed or mixed within a population of cells with differing potentials. Thus going forward, we would submit that experimental models examining mesothelially-derived cell lineages in the gut tube explore timeframes when only definitive mesothelial cells can be identified and labeled. This would entail determining when mesothelial-specific genes are expressed only in the serosal mesothelium and activating Cre expression at that time. Viral- and vital-labeling would again be conducted at those time points. Indeed, our own study [48] should be revisited using temporally-activated Cre expression to ensure that only mesothelial progeny are being labeled. This strategy, in strictest terms, might be effective in identifying descendants of visceral mesothelia. 90,000 what is this? Symptoms Varieties of tumors of the mesothelium. Methods for the treatment of mesothelioma
You should check the information provided here. The decision on the use of a particular substance referred to here is exclusively at the discretion of the attending physician. Be sure to consult your doctor!
Mesothelioma is a malignant formation that forms from cells lining the walls of the secondary cavities of the body:
- Peritoneum, where the organs of the gastrointestinal tract are located.
- Pleura surrounding the lungs.
- Pericardium, inside the cavity of which the heart is located.
- The inner side of the scrotum, where the testicles are located.
The lining is called mesothelium or coelomic epithelium. It is flat, sufficiently smooth and elastic, which allows the internal organs in the middle of the cavity to move freely, without friction (the lungs breathe, the heart beats).
Neoplasms in the mesothelium rarely develop, since almost the only triggering mechanism of carcinogenesis is direct human contact with asbestos (80% of cases).This compound is capable of accumulating in the inner membranes and provoking mutations. Therefore, the problem concerns people working in the extraction of asbestos and the creation of products from it. Moreover, the progression of the tumor begins a long time after contact. Usually more than twenty to thirty years should pass. Every year in the world, only two to three thousand patients are diagnosed. In Ukraine, up to 250 cases of mesothelioma are detected annually.
Most of them suffer from elderly men over 50 years old.They face the disease eight times more often than women.
Among tumors, formations developing in the pleura prevail (89%), in second place in terms of the prevalence of neoplasms in the peritoneum – 9.6%. Other varieties account for less than two percent.
Classification of mesotheliomas
All tumors of this type are divided into:
- Diffuse , in which the cells do not adjoin each other tightly, without forming a clear structure.A tumor without even borders, resembles a thickening on the surface, can spread to large areas. Cancer cells actively penetrate into deeper layers of tissues and grow into neighboring organs. The majority of such tumors (75%) occur.
- Localized, with strict boundaries. They resemble a light, protruding node, consisting of cells similar to epithelial cells. Such a formation is considered more benign, since it does not give metastases and does not affect neighboring organs.
Depending on the structure of cells in the diffuse type of mesothelioma, several subspecies are distinguished:
- Epithelioid tumors . The most common (54% of all cases). They spread over the surface of the mesothelium. Cells are similar in structure to epithelial cells.
- Sarcomatoid. Their structures quickly grow into internal connective tissues and are similar to them in the appearance of cells.
- Desmoplastic .More often formed in the abdominal cavity. Cancer elements are rounded. A dense connective tissue edging is formed around the tumor.
- Mixed (biphasic), consisting of cells of the first two groups. They are quite common – in 25% of patients.
There are also very rare forms that today are almost never diagnosed. These are papillary mesothelioma and adenomatous tumor. They are considered benign because they do not metastasize.
Main symptoms
The tumor grows slowly, so the symptoms do not appear immediately. Sometimes three to five years pass before the first clinical manifestations. As a result, the formation is detected mainly in the late stages, which reduces the survival rate. After diagnosis, life expectancy does not exceed two years.
As the first symptom, 70% of patients report pain. It is dull in nature, it is difficult to determine the exact place of its localization.Further in the affected area, fluid begins to accumulate. This is due to the fact that all mesotheliomas are capable of producing mucus. Weight loss and an increase in body temperature are observed only in 30% of cancer patients.
The rest of the symptoms are more specific and depend on the location of the focus.
Pleural tumors are characterized by cough, bloody sputum, joint swelling and thickening of the fingers. As the disease progresses, hoarseness of the voice appears, compression of the vena cava, which disrupts blood circulation in the head and neck.Fluid buildup causes shortness of breath.
With a tumor of the peritoneum , there is bloating, nausea, and upset stools. The abdomen increases in size as mucous secretions accumulate. Swelling of the legs occurs due to the squeezing of the vessels by the enlarged abdomen.
Damage to the pericardium is initially limited to pain and cardiac dysfunctions similar to other heart diseases. And after the accumulation of fluid, suffocation can develop, significant circulatory disorders.
Stages
The progression of the disease occurs slowly in time, but aggressively in the capture of surrounding tissues. The cells of the formation germinate into neighboring tissues at the first stages, and at the third stage, multiple metastases are observed. The most accurate description of the stages exists only for pleural cancer.
- At the first stage, the lining of the cavity is affected.
- Next, the cancer affects the lung tissue with which it most often comes into contact.
- The mass affects the bursa of the heart and the mediastinum (tissues located in the center of the sternum in front).
- Cancer affects the intercostal muscles, surrounding organs and blood vessels.
Other mesotheliomas proceed according to a similar pattern, spreading to the surrounding tissues and giving metastases, starting from the third stage.
Diagnostics and treatment
The most common diagnostic methods are:
- X-ray , which shows if there are wall thickenings and fluid in the cavity.
- Computed tomography , which determines how much the tumor has spread.
- Thoracoscopy and laparoscopy with biopsy . At the same time, an apparatus is inserted into a special incision, with the help of which the doctor examines the diseased cavity from the inside and takes the tissue for analysis.
Mesothelioma is easily confused with other diseases. Even when symptoms become overt, they resemble inflammation of the lining membranes, resemble those of a sarcoma, or are mistaken for metastases from other tumors.Only the examination of tissue samples helps to determine the type of cancer. But even then, the correct diagnosis is made only in 80% of cases.
Unfortunately, today there are no effective methods of treating mesothelioma.
The operation is performed only by 10% of patients, those who are diagnosed with a localized tumor. In 60% of cases, complications appear after resection, and only 35% of those operated on live for more than two years.
Chemotherapy gives temporary improvement and only for 48% of patients.For its implementation, modern cytostatics are used – drugs that stop the division of tumor cells and provoke their death. To reduce the activity of mucus secretion by a tumor, cytostatics can be injected directly into the diseased cavity.
Irradiation is used to relieve symptoms in order to improve the quality of life of a cancer patient. Radiotherapy prolongs life by about one year.
The best results with a 45% survival rate are obtained by combining all three therapies, starting with surgery, followed by six courses of chemotherapy and final radiation.
Forecast
The most aggressive tumor with a poor prognosis is sarcomatoid mesothelioma. For other types of formations, there are factors that are considered unfavorable and when they are detected, only 14% of patients step over the annual survival boundary. These factors include:
- Decrease in hemoglobin level and significant increase in leukocytes.
- Damage to the lymph nodes.
- Metastases.
- Low index on the Karnofsky scale – helps to determine the patient’s condition depending on his preservation of mobility and activity.The index is considered low if a person cannot take care of himself and spends half of the day in bed exhausted.
If these signs are not present, then the prognosis improves and the patient survives 42% of cases.
90,000 Mesothelial cysts of the pelvis and abdominal cavity: a case report | Popov
1. Chirkov R.N., Vakarchuk I.V. Origin of extraorgan cysts of the retroperitoneal space.Topical issues in science and practice. Tver, 2018. P. 13–19.
2. Branca G., Ieni A., Barresi V., Versaci A. A retroperitoneal cyst with unusual urinary histogenesis: clinical and immunomorphological characteristics. Int Med Case Rep J 2010; 3: 81-5.
3. Alzaraa A., Mousa H., Dickens P. et al. Idiopathic benign retroperitoneal cyst: a case report.J Med Case Rep 2008; 2 (1): 1-4. DOI: 10.1186 / 1752-1947-2-43.
4. Yohendran J., Dias M. M., Eckstein R., Wilson T. Benign retroperitoneal cyst of Mullerian type. Asian J Surg 2004; 27 (4): 333-5.
5. Haddadin W.J., Reid R., Jindal R.M. A retroperitoneal bronchogenic cyst: a rare cause of a mass in the adrenal region. J Clin Pathol 2001; 54 (10): 801-2.
6. Sigua B.V., Zemlyanoy V.P., Kozobin A.A. et al. Surgical tactics in patients with multichamber post-traumatic cyst of the retroperitoneal space. Journal them. N.V. Sklifosovsky Research Institute for Emergency Medicine 2017; 6 (2): 162-5.
7. Antonov A.V. Retroperitoneal fluid formations: diagnosis and treatment. Urological Bulletin 2012; 2 (4): 32–41.
8. Veldhuis W.B., Akin O., Goldman D. et al. Peritoneal inclusion cysts: clinical characteristics and imaging features. Eur Radiol 2013; 23 (4): 1167-74. DOI: 10.1007 / s00330-012-2695-8.
9. Singh A., Sehgal A., Mohan H. Multilocular peritoneal inclusion cyst mimicking an ovarian tumor: A case report. J Midlife Health 2015; 6 (1): 39-40.DOI: 10.4103 / 0976-7800.153648.
10. Pernick N. Benign cystic mesothelioma. PathologyOutlines.com website. Available at: http://www.pathologyoutlines.com/topic/smallbowelbenigncysticmesothelioma.html.
SCVP Endomyocardial Biopsy Tutorial
Histological findings in the absence of a rejection reaction
This section of the manual will determine the number of histological findings in the absence of a rejection reaction.
and the conclusions that can be drawn from these biopsies.Some of these results are general and some are extremely rare. Many of these findings can be easily confused with rejection reactions, and it is important that these errors do not occur.
Quilty Effect
Endomyocardial biopsies in ~ 50% of patients with cardiac allografts may have a focal, dense collection of endocardial lymphocytes that may extend to adjacent areas of the myocardium.These general changes are called the Quilty effect (formerly the Quilty lesion, named after the first patient in whom they were described). These foci are composed of a mixture of B-lymphocytes and T-lymphocytes, and sometimes dendritic cells. Also, sometimes small vascular channels (capillaries) can be noted, in some inflammatory infiltrates.
The pathogenesis of Quilty’s effect is unknown, but hypotheses include an immunosuppressive regimen based on cyclosporin, an idiosyncratic response to cyclosporin A, and concomitant Epstein-Barr viral infection.Quilty effect is not observed in the heart in patients who received cyclosporine for other organ transplants (eg, kidney, liver). Many scientists have investigated the relationship between the Quilty effect and the degree of cellular rejection, viral infection, AMR, and / or the development of graft vasculopathy. Research both support and refute the link to these findings by making this area open. In general, there is no consensus on the relationship between the Quilty effect and key histopathological findings.
Quilty effect can only be in the endocardium, or it can spread to adjacent areas of the myocardium. Some of the major effects of Quilty are distributed among the main myocytes and result in peri-Quilty myocytic damage. This simulated rejection reaction is considered a common etiology for ISHLT-1990 grade 2 rejection. In this case, a distinction from acute cellular rejection must be made. This may require several additional sections to establish that there is an endocardial component (although acute cellular rejection may also extend to the endocardium).If these changes are present, small vessels or capillaries in the inflammatory infiltrate can be very helpful in recognizing the Quilty effect. In addition, the stroma among myocytes in the Quilty effect may appear to be more fibrous.
In some cases, lymphocyte immunoperoxidase assay may be necessary, in which case the Quilty lesion will have B and T lymphocytes and macrophages, unlike acute cellular rejection, in which T cells are predominant, CD21 staining can also be used.This is a strong positive marker for the Quilty effect. Negative CD21 staining is not prognostic.
If the Quilty effect occurs in other parts of the biopsy, it may be more likely that the myocardial inflammation is also Quilty, although an acute cellular rejection reaction cannot be completely ruled out. After the Quilty effect heals, small scars remain. Quilty scars tend to have more linear boundaries, in contrast to previous biopsies, which are more irregular.
Ischemic injury
Ischemic injury (from transplant) is usually noted in biopsies taken within the first 6 weeks after transplant, but may be present for up to 3 months. This process can be difficult to distinguish from acute cellular rejection. Due to ischemia of the heart, when a donor heart is transplanted to a recipient, a certain number of myocytes will die. Dead myocytes, as a rule, are located together, large or small islets).In the initial biopsy, changes in myocytes can be very superficial and only eosinophilia of the cytoplasm is noticeable and anucleated cells are visible. Dead myocytes will stain very strongly with C4d (immunohistochemically or by immunofluorescence). This should not be confused with antibody-induced rejection. Damaged myocytes can be seen, but the absence of lymphocytic infiltrate is pronounced. Because of the strong immunosuppression, inflammation may not be seen in the early stages of the process.
In general, the maximum inflammatory response is present 2-4 weeks after transplant.This is primarily a macrophage-mediated response. Inflammation can be extensive and associated with damage to myocytes. The key feature to distinguish it from acute cellular rejection is a mixed inflammatory response, not just lymphocytic infiltration. Ischemic damage can also have a “dirty” interstitial background. When there are signs of both acute and ischemic damage in the same biopsy, making the distinction difficult.
This is a very common mistake due to the dual process, but since most patients return for a repeat biopsy within a week or two, care must be taken.After the first month, most ischemic lesions appear as a typical resolving small infarction with pigmented macrophages, loose connective tissue, and regional loss of myocytes. This is not easily confused with acute cellular rejection, but it is difficult to distinguish from the previous biopsy site.
Histological changes at the biopsy site and blood clots
Due to the architecture of the right ventricle, the biotome often falls into the site from a previous biopsy and this should be noted.The histological changes at the previous biopsy site vary depending on the interval between the current biopsy and the previous biopsy. The most recent biopsy may result in a focal endomyocardial defect with fibrin deposition or organization of a thrombus and some inflammatory cells (macrophages, T and B cells). In the reparative stage, it may consist of granulation tissue, and eventually the site of endocardial injury will be visible. Myocytes adjacent to this site will show disorganization and / or irregular hypertrophy.This can manifest itself as myocyte desynchronization. In situations in which patients undergo multiple endomyocardial biopsies, such as heart transplants, the likelihood of obtaining a previous biopsy site has been reported to range from one to seven in nearly 70% of biopsies.
Inflammation at the biopsy site alteration site is not an acute cellular rejection and should not be classified as such by degree. Thus, the biopsy does not reflect the degree of rejection (0R), i.e.to there are fields of inflammation associated with a previous biopsy.
Intravascular lymphocytes
In some patients, filling of lymphatic channels with lymphocytes is noted. The etiology of this is unknown. This fact is not associated with an acute cellular rejection reaction. Adipose tissue and mesothelium cells.
Adipose tissue and mesothelial cells
Adipose tissue is a normal, minor component of the myocardium, often adjacent to large arterioles.Sometimes a large number of adipocytes, or overt adipose tissue is observed in biotats. Often, they have small portions of a non-compact myocardium. These samples were taken from the free wall of the right ventricle and, because of their location, they are not suitable for the assessment of acute rejection. Sometimes the presence of adipose tissue is more ominous. If there are strips of mesothelium either with adipose tissue or separately in a block, or when small nerves are visible in the adipose tissue, this indicates that the tissue is derived from the epicardium.This means a perforation of the right ventricle and the attending physician should be urgently informed about this fact due to the risk of significant hemopericardium. In most patients, the pericardium is attached to the heart, with the result that the risk of fatal hemopericardium is significantly reduced compared to that in a non-transplanted patient.
Calcification of myocytes
Calcification of myocytes is an extremely rare finding in heart transplants.The etiology is considered to be transient hypercalcemia during the transplantation event with metastatic calcification of myocytes. This can also be seen at the sites of previous biopsies. Calcification is most likely to occur in the setting of acute or chronic renal failure. Histologically, this looks like calcification of myocytes, either individually or in groups. Increased fibrosis can occur in these areas, and inflammation is not typical. Although extensive cardiac calcification can adversely affect heart function, it is not associated with an acute cellular rejection response.
Recurrence of the disease
Several pathological processes that lead to heart failure have been reported to recur in a transplanted heart. These include sarcoidosis, myocarditis, amyloidosis, Chagas disease, and various other diseases. The pathologist should be aware of any of the reasons why the patient received a heart transplant and be aware of the recurrence of these diseases. Sarcoidosis and myocarditis can be particularly similar to acute cellular rejection.
Infection
Infection is very rarely found on endomyocardial biopsy, although recipients are at increased risk of infection due to immunosuppression. Cytomegalovirus (CMV) may be present, but CMV status is generally better, determined serologically (only if the recipient is initially CMV negative before transplantation) or in other tissues (eg, the esophagus). However, the presence of viral inclusions can be determined on biopsy material.CMV or other viral immunohistochemical stains can be obtained. If infection is present, the information should be brought to the attention of the physician, but should not be confused with acute cellular rejection. Toxoplasmosis can also be seen in a transplanted heart. Bradyzoites can fill myocytes without significant inflammatory response. It can also be detected using immunohistochemistry.
Post-transplant lymphoproliferative disorder (PTLD)
Although after transplantation, lymphoproliferative disorders
(PTLD) occur in a fairly high number of cases (up to 6%) of patients after transplantation, this is extremely rare, this diagnosis is made on the basis of endomyocardial biopsy.Histologically, this will manifest as an accumulation of B-lymphocytes mimicking an acute cellular rejection response. These cases are usually EBV positive, so an EBV test and immunohistochemistry (CD20) is warranted in suspected PTLD cases. The presence of a significant number of plasma cells in the biopsy is uncommon and may be a plasmacytoid variant of PTLD. Rarely, T cell lymphomas also cause PTLD.
Foreign body
Small clumps of foreign giant cells can be seen in the endocardium in recipients who have had repeat biopsies.They may contain refractile material, most likely fragments of cotton fiber that was used to wipe the biotome between biopsies. Fragments of the tricuspid valve and liver fragments.
Tricuspid valve Fragments and fragments of the liver
About 12% of recipients develop tricuspid murmur after transplantation.The most common cause of this is congestive heart failure, annular enlargement, or pulmonary hypertension. Rarely, this is due to the accidental rupture of the valve or chord by the biotome cusp as it passes through the opening of the tricuspid valve. If this is visible on a biopsy, the attending physician should be informed. A sufficiently large defect can significantly affect the function of the graft. A flap or chord fragment will essentially be a narrow strip of fibrous tissue with elastic fibers horizontally with the long axis of the material.Endothelial cells can be viewed from two sides, as opposed to a piece of thickened endocardium, although this distinction can be very difficult to make.
It is extremely rare that a biotome can pass from the superior vena cava to the inferior vena cava, without clinical signs, and a biopsy can be taken from the liver, and not from the heart. Thus, liver tissue on the biopsy specimen may come from the patient or from possible contamination (pick-up tissue) in a poorly designed histology laboratory.
The Society Information for the Cardiovascular Pathology website is provided with the understanding that the Society does not provide medical advice or advice. You should not rely on the information provided here to substitute for consultation with qualified health care professionals to meet your individual health needs.
Pleural mesothelium in case of damage and regeneration pathways (literature review) Text of a scientific article in the specialty “Fundamental medicine”
UDC 611-018.7: 616.25-001] -003.93
MESOTHELIUM OF THE PLEURA IN DAMAGE AND WAYS OF REGENERATION (LITERATURE REVIEW)
D.A. Semenov, S.S. Tseluiko
Amur State Medical Academy of the Ministry of Health and Social Development of the Russian Federation, 675000, g.factors and cytokines that mobilize the movement of inflammatory cells into the pleural cavity. Inflammation of the pleura can acquire a chronic course: scarring of granulations occurs, fibrinous overlays are organized on the surface of the lung and on the parietal pleura. Ultimately, a thick, rigid wall of the residual cavity is formed, cicatricial septa, pneumosclerosis, pneumocirrhosis of the adjacent part of the lung are formed. The reaction of the pleural mesothelium after injury is a multifactorial process in which coagulation can occur, the formation of adhesions with the development of fibrosis and subsequent obliteration of the pleural cavity.
Keywords: mesothelium, mesotechial wound, regeneration, acute and chronic inflammation, pleurodesis, adhesions.
SUMMARY
MES OTHELIUM OF PLEURA AT INJURY AND NEOGENESIS WAYS (REVIEW)
D.A.Semenov, S.S. Tseluyko
Amur State Medical Academy, 95 Gor’kogo Str., Blagoveshchensk, 675000, Russian Federation
The morphofunctional state of mesothelium allows
to evaluate its regenerative ability. Mesothelial cells and extracellular matrix play a regulatory role in the compensate ry-adaptive reactions of the pleural cavity. Mesothelial cells at pleural injury lose their inter-cell connections, are separated, take the morphology of fibroblasts and cover the surface of pleura.Mesotheliocy-tes create numerous factors and cytokines mobilizing the movement of inflammatory cells into the pleural cavity. The inflammation of the pleura can take a chronic course: there happens granulation scarring, fibrinoge-nous layers are subject to organization on the surface of the lung and on the parietal pleura. In the end, there is a thick, rigid wall of the residual cavity created, scarry walls, pneumosclerosis and pneumocirrhosis of
the adjacent part of the lung are formed.The reaction of pleura mesothelium after the injury is a multiple-factor process under which there can be coagulation, the formation of adhesions with the development of fibrosis and a subsequent obliteration of the pleural cavity.
Key words: mesothelium, mesothelial injury, neogenesis, an acute and chronic inflammation, pleurodesis, adhesions.
The surface of the pleural layers is covered with mesothelium, located on the basement membrane and connective tissue fibrous base, consisting of 3-4 layers.The surface of the pleura is smooth and rather transparent. The parietal pleura occupies a large area compared to the visceral pleura. Mesothelial cells (MC) have a thickness of about 4 μm, are connected at the surface of the pleura by tight joints, in the basal part of the cells they are connected in the form of an adhesive intercellular contact [13]. On the surface of the MC there are microvilli, which are unevenly distributed over the surface of the pleura (Fig. 1, 2). They increase the surface area of metabolic activity and are involved in phagocytosis.The microvilli are 3-5 µm in length and 80 to 90 nm in width.
4 layers are histologically distinguished in the pleura: mesothelium, thin submesothelial collagen layer, superficial elastic layer, deep fibroelastic (ethmoid) layer, which contains blood vessels and nerves [31]. The thickness of the submesothelial interstitium of both the parietal and visceral pleura is approximately 20 µm. However, the thickness of the pleural layers themselves varies considerably.The parietal pleura is 5 times thicker than the visceral. In addition, it contains lymphatic stomata (pores, hatches). The features of the parietal diaphragmatic pleura include transphrenic lymphatic connections, which provide fluid flow from the peritoneum to the pleura. On average, the width of the pleural space is 18–20 µm [12]. The high reactivity of mesothelial cells, leading to pronounced de-complexation, the formation of stigmata and their description, creates conditions for free
movements of macrophage and lymphoid cells [16].
Mesothelium – slowly renewing cells with mitosis of 0.16-0.5% of cells [38]. Mesothelial regeneration involves the migration of free emerging cells from the serous fluid to the surface [21]. Although MCs are of mesodermal origin, they express features of both epithelial and mesenchymal phenotypes. In addition, the new mesothelium
is restored through centripetal ingrowth of cells from the edge and from the free-floating aggregate of the presence of cells in the serous fluid.MK can transform into myofibroblasts and, possibly, smooth muscle cells [16]. The mesothelial “stem” cell has not been identified, but studies suggest that a mesothelial cell progenitor exists [39]. MCs selected for tubular constructs were used for vascular replacements and grafts to connect the dissected nerve fibers. These data prove that MK is able to “switch” between different cell phenotypes depending on the local environment.Clonal analysis was performed along with cell labeling and tracking studies to determine the true existence of the mesothelial stem cell [27].
Fig. 1. Visceral pleura of the rat (data of the authors). Mesothelial cells lie on the basement membrane, which is clearly visible. There are microvilli on the surface of the mesothelial cells. Color: methylene blue. Magnification: 1350.
Fig.2. Visceral pleura of the rat (data of the authors). Fragment of a mesothelial cell with microvilli extending from it. Mitochondria, a developed endoplasmic reticulum, a Golgi complex, and pinocytic microvesicles are visible. Magnification: 10000.
MK secrete macromolecular components of the extracellular matrix and organize it into a mature matrix, phagocytosis particles, fibrinolytic and procoagulant factors, neutrophil and monocyte chemotaxis factors, which may be important for the mobilization of inflammatory cells into the pleural space
cavity [14, 21, 30, 41].MK produce cytokines such as transforming growth factor P (TS1G-P), epidermal and platelet growth factors, which play an important role in the development of pleural inflammation and the formation of fibrosis [34]. MK synthesize the colony-stimulating factor of macrophage-granulocytes for the proliferation and differentiation of granulocytes and monocytes, which enhances the phagocytic and cytotoxic activity of granulocytes and eosinophils. The migration of neutrophils into the pleural cavity is carried out under the influence of some chemokines, in particular, interleukin (IL)
1, 6, 8.The site of IL-8 synthesis is the MCs and their villi involved in the inflammatory process. It is considered as a biomarker in the differential diagnosis of inflammatory and carcinogenic processes [10]. Mesothelial cells contain vimentin, cytokeratin, 1CAM-1 (type 1 intercellular adhesion molecule), and C 044.1CAM-1 molecule was enhanced by the action of tumor necrosis factor (Tyr-a. 100 u / t1). The use of Tyr-a in mesothelial cells significantly increased their affinity for peritoneal mononuclear leukocytes, while pretreatment with TOB-P (2 ng / ml) prevents the formation of adhesions.Culturing for 3 weeks resulted in proliferation, differentiation, and monocytes / macrophages on the mesothelial surface [37].
MK and the extracellular matrix play a regulatory role during the inflammatory-reconstructive reaction of serous membranes, and integrins serve as cellular receptors for these processes [5, 45]. The pleural MV has a critical role in post-wound mesothelium repair through its ability to produce connective tissue macromolecules.For this, rat pleural mesothelial cells were exposed to various combinations of Tyr-a. IL-1, interferon-y and nitric oxide within 24-48 hours. Under these conditions, collagen production was significantly slowed down by the lipopolysaccharide cytokine. Collagen inhibition corresponded to the dynamics of increased nitric oxide production of nitric oxide. The latter can play the role of a mediator that activates collagen production in severe pleural inflammation [40]. To increase the viability of the mesothelial cell, synthetic ebselen was used at a dose of 10–40 cM Eb, which revealed its effectiveness as a cytoprotective agent [26].The localization of the spreading subsurface accumulations in visceral pleural inflammation revealed migrating MCs and remnants of the superficial mesothelium within the organizing fibrous serous membrane during healing. Subsurface mesothelial cell proliferation is unique for hamsters, rats [29], and humans [28].
With pleural injuries, MCs lose intercellular connections, separate, and take on the morphology of fibroblasts, scattering across and covering the surface.As shown by Western blot analysis and im-
by munofluorescence, these cells have a scattering factor receptor (HC1R / 8R). Incubation of mesothelial cells with HC! P / 8P ruptured intercellular connections and caused scattering, which enhanced mesothelial cell migration. In addition, HOP / 8P has shown a significant mitogenic effect and an autocrine role in mesothelial healing [46].
Cyclooxygenase products of cultured human MCs play an important role in inflammatory serous and neoplastic pleural pathologies.The prostanoid product cyclooxygenase 2 is an important component of the inflammatory response in acute and chronic pleural infections [15, 19].
Although acute mesothelial wound has been well researched in models, there is no certainty about the mechanisms of origin of mesothelioma. Experimental pathologists have used observations of mesothelial healing to study the theory of tumor histogenesis. A special mesenchymal “stem cell” exists in the pleura and is the source of new mesothelium during wound healing during malignancy.This stem cell was called a multipotential subserous cell [20, 25]. The second theory suggests that MC are responsible for the renewal of the mesothelium in the wound process in the same way as during malignancy [48]. Superficial mesothelial cells have the ability to proliferate in response to a variety of stimuli, and this is a common feature of the early phase of mesothelial healing [47]. Growth factor derived from macrophages is important in proliferation [36]. With hyperplasia of the pleural mesothelium, an increase in its layers occurs, accompanied by inflammatory cells and an increase in the main connective tissue.MC become cuboid in comparison with “smoothed” normal mesothelial cells. In mesothelioma, a sign of proliferation of the mesothelium and the main connective tissue is visible [23]. Effusion and mesothelial proliferation, a sign of cellular atypia, or invasion of the adjacent lung parenchyma are common [43].
Pleurodesis – pleurisy, artificially created by chemical or mechanical action of injected substances (tetracycline, bleomycin, fibrin, mitoxantrone, talc), the purpose of which is to obliterate the pleural cavity to prevent recurrence (usually malignant) of pleural effusion.In this case, an inflammatory reaction occurs, followed by fibrinous effusion into the pleural space and proliferation of fibroblasts. Pleurodesis can be performed during puncture (immediately after fluid evacuation, chemotherapy drugs are injected into the pleural cavity) or with the help of videothoracoscopic equipment (a video camera and instruments are introduced into the pleural cavity for fluid evacuation and subsequent administration of drugs). The balance between the procoagulant and fibrinolytic systems is probably important in determining whether pleurodesis will end [33].In humans, pleurodesis after the use of talc occurs with a decrease in fibrinolytic activity [42].
When tetracycline is administered intrapleurally to rabbits with heparin or urokinase, the number of pleural adhesions is reduced [44].
Interstitial, subpleural and pleural fibrosis occurs in rats exposed to frequent inhalation exposure to mineral fibers [17, 18, 22].The mesothelial reaction to the introduced particulates occurs especially pronounced in the parietal pleura lining the diaphragmatic surface [24]. Purulent inflammation affecting the lung parenchyma can involve the visceral pleura with the attachment of a fibrinous component [7, 35], the formation of serous adhesions, and malignant mesothelioma can develop [38].
According to the authors of [2], three phases should be distinguished in the development of adhesions.During the first phase, the mesothelium and elastic formations degenerate. Special leukocytes and polyblasts play an important role in their destruction. During the second phase, variously differentiated fibroblasts located on both sides of the elastic formations undergo changes. Their connection with the surrounding gaps and the main substance is broken. Fibroblasts take on a different shape and are stained much more intensely. Also exudative polyblasts and lymphocytes that have got here undergo changes.As a result of the transformation of all the above cells, syncytium is formed. The inner part of the commissure is composed of long, narrow, elliptic cells with long oval nuclei. By their processes, they are interconnected and take the form of syncytium. Narrow, dark-colored elements, apparently, should be considered as undifferentiated mesenchymal elements in contrast to the more differentiated outer layer of syncytium. In the third phase, this syncytium is transformed. An intermediate base substance is formed.Cellular territories are transformed into cellular elements with clearly defined outer contours. In this phase, new capillaries appear, which, as experiments have shown, appear for the first time 4-6 days after the operation. Capillaries grow from the edges of the flap to its middle. Initially, as a rule, they are located only in the undifferentiated section of the syncytium, among narrow, intensely colored elements. In the development of capillaries, cells of the undifferentiated adhesion region are of great importance. This section of the syncytium is not only a place for the development of capillaries, but its cells are the elements from which, apparently, the capillary wall is built.Capillary development is the proliferation of endothelial cells located at the end of the capillary.
In case of ineffectiveness of treatment, inflammation of the pleura acquires a chronic course: granulations are scarred, fibrinous overlays are organized on the surface of the lung and on the parietal pleura. A rigid residual cavity is created, in which a purulent process remains, which acquires a chronic form. With the transition of pleural empyema to
the chronic form stabilizes the collapse of the lung, and therefore the treatment of pleural empyema can only be operative [1].Histological examination determines a sharp violation of the blood flow of the microvasculature of the pleura, an increase in the permeability of capillaries, arterioles and venules, the appearance of pronounced plasma exudation and immigration of blood corpuscles. In the cytoplasm of endothelial cells, pronounced edema and ultrastructural disorganization of the cytoskeleton occur, which significantly limits their potential for self-contraction [3]. Increased vascular permeability and disruption of desmosomal contacts between alveolocytes promotes exudation and swelling of the interstitial structures of acini, transendothelial emigration of leukocytes, erythrocytes, platelets and, as a result, leads to the transition of inflammation in the border zone into exudative hemorrhagic pneumonia.Thickening of the walls of the alveoli due to the formation of giant Pirogov-Langhans cells, resorption of surfactant by macrophage cells, as well as spasm of bronchioles, lead to the formation of zones of atelectasis, which alternate with foci of emphysema. Foci of lung necrosis are also found, which are surrounded by purulent-fibrinous exudate. In such zones of purulent-necrotic inflammation and atelectasis, foci of progressive pulmonary fibrosis and sometimes pneumocirrhosis develop [4, 8]. In macrophages, ultrastructural signs of damage to membrane organelles are observed.Fibroblast division and cytodifferentiation are slowed down, ultrastructural signs of collagenogenesis are poorly expressed. As a result of disorganization of stromal-parenchymal relationships, the reparative regeneration of the mesothelium, epithelial cells and other cellular elements of the airways and respiratory parts of the lung is limited. When studying the blood vessels of the inflammation zone, productive endo-, peri- and panvasculitis, aggregation and agglutination of erythrocytes with the formation of fibrin, platelet and leukocyte microthrombi are found, which leads to increased hypoxia and deep metabolic disorders of the lung tissue.The result of these processes is the formation of defective granulation tissue in the defect zone [11]. Inhibition of proliferation and cytodifferentiation of cells of fibroblastic diferon, alternation of focal defective collagenogenesis, repeated necrosis and exacerbation of inflammation lead to a violation of adequate reparative correlations between connective tissue and epithelium and chronicity of the purulent-necrotic process. The relationships between alteration, inflammation, regeneration and fibrosis are disrupted, leading to persistent inflammation.There is a large number of leukocytes with signs of destruction, a high frequency of karyopycnosis of endothelial cells and mesothelial cells, as well as a low level of the number of macrophages and functionally active fibroblasts. DNA-synthetic
becomes low
ability of mesothelium, endothelium and fibroblasts [9]. Such cellular changes lead to the formation in the early stages (in the first three days) of granulation tissue in the defect zone with pronounced areas of necrotic detritus, which subsequently is one of the reasons for the formation of repeated inflammation and activation of alteration processes.Disorders of microcirculatory blood supply associated with damage to arterioles, venules and capillaries, the formation of microthrombi in them also persist. The latter leads to prolonged tissue hypoxia, a decrease in the oxidative processes of intracellular respiration, a change in the permeability of cell membranes and the accumulation of water inside cells. The combination of these processes leads to a slowdown in the transition of inflammation to the proliferative stage, undulating sclerosis and lysis of granulation and cicatricial connective tissue, disruption of adequate reparative processes in connective tissue and epithelium.Ultimately, the process turns into a chronic stage, with the formation of a thick, rigid wall of the residual cavity, the formation of multiple cicatricial septa in the cavity itself, severe pneumosclerosis and pneumocirrhosis of the adjacent part of the lung [6].
According to the authors of [32], minimizing damage to the mesothelium by preventing desiccation, careful handling of tissues, adding oxygen and cooling reduce the formation of adhesions by 25%.The addition of calcium channel blockers, phospholipids and dexamethasone, anti-angiogenic monoclonal antibodies and the effect on fibroblasts brings the efficiency of adhesion prevention closer to 100%.
REFERENCES
1. The role of invasive methods in the diagnosis and treatment of pleurisy / NV Vlasova [et al.] // Klin, microbiol. and antimicrobial, chemotherapy. 2003. Vol.5. Application.
S.14.
2. Glotov V.A. Cell and tissue engineering of the endothelium. Formation in culture of endothelium in vitro of functioning self-developing capillary networks. Experimental approaches // Mathematical morphology: electronic mathematical and medical biological journal. 1997. Vol.2, No. 1. S.23-58 ..
3. Danzig P.P., Skibsky I.M., Levin N.F. Modern clinical and morphological characteristics of acute purulent-destructive lung diseases // Pulmonology.2000. No. 2. S. 19-22.
4. Kabanov A. N., Sitko L. A. Treatment of pleural empyema // Grudn. hir. 1985. No. 4. S.27-31.
5. Histophysiology of rat lungs under cold exposure against the background of arabinogalactan administration /
ON Lee [et al.] // Bul. fiziol. and patol. breathing. 2011. Issue 39. S.40-42.
6.Neverov A.N., Tretyakov A.A., Stadnikov A.A. Microbiological and morphofunctional aspects of the use of sporobacterin in the treatment of acute pleural empyema // Vestn. OSU. 2006.
No. 6. S. 132-138.
7. Samsonov K.V. Local detoxification in the treatment of acute pleural empyema // Bul. fiziol. and patol. breathing. 2007. Issue 27. S.36-37.
8.Samsonov K.V. The volumetric rate of lymph flow as an indicator of endotoxicosis in purulent-necrotic diseases of the lungs and pleura in an experiment on animals // Bul. fiziol. and patol. breathing. 2011. Issue 39. S.43-45.
9. Tseimakh EA Treatment of acute pleural empyema and pyopneumothorax // Grudn. and heart-vessel, chir. 1999. No. 1. S.51-54.
10. Chuchalin AG Pleura: pathophysiological and clinical aspects // Ter.arch. 1999. No. 3. S.5-9.
11. Local treatment of acute pleural empyema and pyopneumothorax / Ya.N. Shoikhet [et al.] // Pulmonology. 2002. No. 3. S.47-51.
12. Agostoni E., D’angelo E. Thickness and pressure of the pleural liquid at various heights and with various hydrothoraces // Respir. Physiol. 1969. Vol.6, No. 3. P.330-342.
13.Agostoni E. Mechanics of the pleural space // Physiol. Rev. 1972. Vol.52, No. 1. P.57-128.
14. Pleural mesothelial cell expression of C-C (monocyte chemotactic peptide) and C-X-C (interleukin-8) chemokines / Antony V.B. [et al.] // Am. J. Respir. Cell Mol. Biol. 1995. Vol. 12, no. 6. P.581-588.
15. Baer A.N., Green F.A. Cyclooxygenase activity of cultured human mesothelial cells // Prostaglandins.1993. Vol.46, No. 1. P.37-49.
16. Bakalos D., Constantakis N., Tsicricas T. Distinction of mononuclear macrophages from mesothelial cells in pleural and peritoneal effusions. // Acta Cytol. 1974. Vol. 18, no. 1. P.20-22.
17. Long-term pulmonary responses of three laboratory rodent species to subchronic inhalation of pigmentary titanium dioxide particles / E. Bermudez [et al.] // Toxicol.Sci. 2002. Vol.70, No. 1. P.86-97.
18. Pulmonary responses of mice, rats, and hamsters to subchronic inhalation of ultrafine titanium dioxide particles / E. Bermudez [et al.] // Toxicol. Sci. 2004. Vol. 77, no. 2. P. 347-57.
19. Cho W.S., Chae C. Expression of cyclooxygenase-2 in swine naturally infected with Actinobacillus pleurop-neumoniae // Vet. Pathol. 2003. Vol.40, No. 1. P.25-31.
20. Craighead J.E. Current pathogenetic concepts of diffuse malignant mesothelioma // Hum. Pathol. 1987. Vol. 18, no. 6. P.544-557.
21. Efrati P., Nir E. Morphological and cytochemical investigation of human mesothelial cells from pleural and peritoneal effusions: a light and electron microscopy study // Isr. J. Med. Sci. 1976. Vol. 12, no. 7. P.662-673. ‘
22.Effects of subchronically inhaled carbon black in three species. I. Retention kinetics, lung inflammation, and histopathology / A. Elder [et al.] // Toxicol. Sci. 2005. Vol.88, No. 2. P.614-629.
23. Pleural lesions in Syrian Golden hamsters and Fischer-344 rats following intrapleural instillation of man-made ceramic or glass fibers / J. I. Everitt [et al.] // Toxicol.
Pathol. 1994.Vol.22, no. 3. P.229-236.
24. Comparison of pleural responses of rats and hamsters to subchronic inhalation of refractory ceramic fibers / J. I. Everitt [et al.] // Environ. Health Perspect. 1997. Vol. 105. Suppl. 5. P.1209-13.
25. Studies on the morphological patterns of asbestos induced mesotheliomas in vivo and in vitro / I. P. Gormley [et al.] // Carcinogenesis. 1980. Vol.l, no. 3. P.219-231.
26. Hardej D., Billack B. Ebselen protects brain, skin, lung and blood cells from mechlorethamine toxicity // Toxicol. Ind. Health. 2007. Vol.23, No. 4. P.209-221.
27. Herrick S.E., Mutsaers S.E. Mesothelial progenitor cells and their potential in tissue engineering // Int. J. Biochem. Cell Biol. 2004. Vol.36, No. 4. P.621-642.
28.Herbert A., Gallagher P.J. Pleural biopsy in the diagnosis of malignant mesothelioma // Thorax. 1982. Vol.37, No. 11. P.816-821.
29. Hill R.J., Edwards R.E., Carthew P. Early changes in the pleural mesothelium following intrapleural inoculation of the mineral fiber erionite and subsequent development of mesotheliomas // J. Exp. Pathol. (Oxford) 1990. Vol. 71, No. 1. P. 105-118.
30. Pathways of fibrin turnover of human pleural mesothelial cells in vitro / S.Idell [et al.] // Am. J. Respir. Cell Mol. Biol. 1992. Vol.7, No. 4. P.414-426.
31. Krahl V.E. Anatomy of the mammalian lung // In: W.D. Fenn, H. Rahn (eds.). Handbook of Physiology, Section 3, Respiration. Washington: Am. Physiol. Soc. 1964. Vol.l. P.213-284.
32. Koninckx PR., Molinas R., Binda M.M. Postoperative adhesions and their prevention maintenance of soldering // Rus. J. Hum.Reprod. 2009. Vol.3. P.26-35.
33. Light R.W., Vargas F.S. Pleural sclerosis for the treatment of pneumothorax and pleural effusion // Lung. 1997. Vol. 175, Vol. 4. P.213-223.
34. Light R.W. Pleural effusion // N. Engl. J. Med. 2002. Vol.346, No. 25. P.1971-1977.
35. Lopez A. Respiratory system, thoracic cavity, and pleura // In: M.D. McGavin, J. F. Zachari (eds.). Pathologic basis of veterinary disease. Elsevier, 2007. P. 463-558.
36. Acute injury and regeneration of the mesothelium in response to asbestos fibers / P.A. Maolli [et al.] // Am. J. Pathol. 1987. Vol. 128. P.426-445.
37. Muller J., Yoshida T. Interaction of murine peritoneal leukocytes and mesothelial cells: in vitro model system to survey cellular events on serosal membranes during inflammation // Clin.Immunol. Immunopathol. 1995. Vol. 75, no. 3.P231-238.
38. Mutsaers S.E. The mesothelial cell // Int. J. Biochem. Cell Biol. 2004. Vol.36. # 1. P.9-16.
39. Mutsaers S. Plasticity of the mesothelium: A stem cell in the midst? // Lung Cancer. 2006. Vol.54. Suppl. 1. P.3-4.
40. Owens M.W., Milligan S.A., Grisham M.B. Inhibition of pleural mesothelial cell collagen synthesis by nitric oxide // Free Radic.Biol. Med. 1996. Vol.21. Issue 5.P. 601-607.
41. Role of pleural mesothelial cells in the production of the submesothelial connective tissue matrix of lung /
S. I. Rennard [et al.] // Am. Rev. Respir. Dis. 1984. Vol. 130, No. 2. P.267-274.
42. Failure of talc pleurodesis is associated with increased pleural fibrinolysis / F.Rodriguez-Panadero [et al.] // Am. J. Respir. Crit. Care Med. 1995. Vol. 151, No. 3 (Pt.l). P.785-790.
43. Proliferative and nonproliferative lesions of the rat
and mouse respiratory tract / R. Renne [et al.] // Toxicol. Pathol. 2009. Vol.37, No. 7 (Suppl.). 5S-73S.
44. Effects of intrapleural heparin or urokinase on the extent of tetracycline-induced pleural disease / C.Strange [et al.] // Am. J. Respir. Crit. Care Med. 1995. Vol. 151, No. 2 (Pt.l). P.508-515.
45. Expression and function of pi and P3 integrins of human mesothelial cells in vitro / L. Tietze [et al.]. // Exp. Mol. Pathol. 1999. Vol. 66, no. 2. P.131-139.
46. HGF / SF induces mesothelial cell migration and proliferation by autocrine and paracrine pathways / R. Warn [et al.] // Exp. Cell. Res.2001. Vol. 267. Issue 2. P.258-266.
47. Whitaker D, Papademitriou J. Mesothelial healing: morphological and kinetic investigations // J. Pathol. 1985. Vol. 145, No. 2. P.159-175.
48. The pathobiology of mesothelium / Whitaker D. [et al.] // In: D. W. Henderson, K.B. Shilkin, S. L. P. Langlois, D. Whitaker (eds.). Malignant Mesothelioma. New York: Hemisph. Pub. Corp. 1992. P. 25-56.
REFERENCES
1.Vlasova N.V., Girinchir V.K. Semendyaev S.S. Shinkar ‘M.B. Klinicheskaya mikrobiologiya i antimikrob-naya khimioterapiya 2003; 5 (Suppl.): 14.
2. Glotov V.A. Matematicheskaya morfologiya: elek-tronnyy matematicheskiy i mediko-biologicheskiy zhurnaX 1997; 2 (1): 23-58.
3. Dantsig 1.1., Skibskiy I.M., Levin N.F. PuV-monologiya 2000; 2: 19-22.
4.-five.
9. Tseymakh E.A. Grudnaya i serdechno-sosudistaya khirurgiya 1999; 1: 51-54.
10. Chuchalin A.G. Terapevticheskii arkhiv 1999; 3: 5-
9.
11. Shoykhet Ya.N., Tseymakh E.A., Mal’chenko T.D., Tryankina S.A., Roshchev I.P., Sedov V.K., Limanov A.E. Pul’monologiya 2002; 3: 47-51.
12. Agostoni E., D’angelo E. Thickness and pressure of the pleural liquid at various heights and with various hydrothoraces Respir. Physiol. 1969; 6 (3): 330-342.
13. Agostoni E. Mechanics of the pleural space Physiol. Rev. 1972; 52 (1): 57-l28.
14. Antony V.B., Hott J.W., Kunkel S.L., Godbey S.W., Burdick M.D., Strieter R.M. Pleural mesothelial cell expression of C-C (monocyte chemotactic peptide) and C-X-C (interleukin-8) chemokines Am. J. Respir. Cell Mol. Biol. 1995; 12 (6): 581-588.
15. Baer A.N., Green F.A. Cyclooxygenase activity of cultured human mesothelial cells. Prostaglandins 1993; 46 (l): 37-49.
16. Bakalos D., Constantakis N., Tsicricas T. Distinction of mononuclear macrophages from mesothelial cells in pleural and peritoneal effusions.Acta Cytol. 1974; 18 (l): 20-22.
17. Bermudez E., Mangum J.B., Asgharian B., Wong B.A., Reverdy E.E., Janszen D.B., Hext P.M., Warheit
D.B., Everitt J.I. Long-term pulmonary responses of three laboratory rodent species to subchronic inhalation of pigmentary titanium dioxide particles. Toxicol. Sci. 2002; 70 (l): 86-97.
18.Bermudez E., Mangum J.B., Wong B.A., Asgharian B., Hext P.M., Warheit D.B., Everitt J.I. Pulmonary responses of mice, rats, and hamsters to subchronic inhalation of ultrafine titanium dioxide particles. Toxicol. Sci. 2004; 77 (2): 347-357.
19. Cho W.S., Chae C. Expression of cyclooxygenase-2 in swine naturally infected with Actinobacillus pleurop-neumoniae Vet. Pathol. 2003; 40 (1): 25-31.
20.Craighead J.E. Current pathogenetic concepts of diffuse malignant mesothelioma. Hum. Pathol. 1987; 18 (6): 544-557.
21. Efrati P., Nir E. Morphological and cytochemical investigation of human mesothelial cells from pleural and peritoneal effusions: a light and electron microscopy study Isr. J. Med. Sci. 1976; 12 (7): 662-673.
22. Elder A., Gelein R., Finkelstein J.N., Driscoll K.E., Harkema J., Oberdorster G. Effects of subchronically inhaled carbon black in three species. I. Retention kinetics, lung inflammation, and histopathology. Toxicol. Sci. 2005; 88 (2): 614-629.
23. Everitt J.I., Bermudez E., Mangum J.B., Wong B., Moss O.R., Janszen D., Rutten A.A. Pleural lesions in Syrian Golden hamsters and Fischer-344 rats following intrapleural instillation of man-made ceramic or glass fibers. Toxicol. Pathol. 1994; 22 (3): 229-236.
24. Everitt J.I., Gelzleichter T.R., Bermudez E., Mangum J.B., Wong B.A., Janszen D.B., Moss O.R. Comparison of pleural responses of rats and hamsters to subchronic inhalation of refractory ceramic fibers Environ. Health Perspect. 1997; 105 (Suppl. 5): 1209-1213.
25. Gormley I. P, Bolton R. E., Brown G., Davis J. M., Donaldson K. Studies on the morphological patterns of asbestos induced mesotheliomas in vivo and in vitro.Carcinogenesis 1980; 1 (3): 219-231.
26. Hardej D, Billack B. Ebselen protects brain, skin, lung and blood cells from mechlorethamine toxicity Toxicol. Ind. Health 2007; 23 (4): 209-221.
27. Herrick S.E., Mutsaers S.E. Mesothelial progenitor cells and their potential in tissue engineering. Int. J. Biochem. Cell Biol. 2004; 36 (4): 621-642.
28.Herbert A., Gallagher PJ. Pleural biopsy in the di-
agnosis of malignant mesothelioma. Thorax 1982; 37 (11): 816-821.
29. Hill R.J., Edwards R.E., Carthew R Early changes in the pleural mesothelium following intrapleural inoculation of the mineral fiber erionite and subsequent development of mesotheliomas. J. Exp. Pathol. (Oxford) 1990; 71 (1): 105-118.
30.Idell S., Zwieb C., Kumar A., Koenig K.B., Johnson A.R. Pathways of fibrin turnover of human pleural mesothelial cells in vitro. Am. J. Respir. Cell Mol. Biol. 1992; 7 (4): 414-426.
31. Krahl V.E. Anatomy of the mammalian lung. In: Fenn W.D., Rahn H., editors. Handbook of Physiology, Section 3, Respiration, Vol.l. Washington: Am. Physiol. Soc .; 1964: pp. 213-284.
32. Koninckx PR., Molinas R., Binda M.M. Postoperative adhesions and their prevention maintenance of soldering. Rus. J. Hum. Reprod. 2009; 3: 26-35.
33. Light R.W., Vargas F.S. Pleural sclerosis for the treatment of pneumothorax and pleural effusion. Lung 1997; 175 (4): 213-223.
34. Light R.W. Pleural effusion. N. Engl. J. Med. 2002; 346 (25): 1971-1977.
35.Lopez A. Respiratory system, thoracic cavity, and pleura. In: McGavin M. D., Zachari J. F., editors. Pathologic basis of veterinary disease. Elsevier; 2007: pp.463-558 ..
36. Maolli P.A., MacDonald J.L., Goodlick L.A., Kane
A.B. Acute injury and regeneration of the mesothelium in response to asbestos fibers. Am. J. Pathol. 1987; 128: 426445.
37.Muller J., Yoshida T. Interaction of murine peritoneal leukocytes and mesothelial cells: in vitro model system to survey cellular events on serosal membranes during inflammation. Clin. Immunol. Immunopathol. 1995; 75 (3): 231-238.
38. Mutsaers S.E. The mesothelial cell. Int. J. Biochem. Cell Biol. 2004; 36 (1): 9-16.
39. Mutsaers S. Plasticity of the mesothelium: A stem cell in the midst? Lung Cancer 2006; 54 (Suppl.l): 3-4.
40. Owens M.W., Milligan S.A., Grisham M.B. Inhi-
bition of pleural mesothelial cell collagen synthesis by nitric oxide. Free Rad. Biol. Med. 1996; 21 (5): 601-607.
41. Rennard S.I., Jaurand M.C., Bignon J., Kawanami O., Ferrans V.J., Davidson J., Crystal R.G. Role of pleural mesothelial cells in the production of the submesothelial connective tissue matrix of lung.Am. Rev. Respir. Dis. 1984; 130 (2): 267-274.
42. Rodriguez-Panadero F., Segado A., Martin Juan J., Ayerbe R., Torres Garcia I., Castillo J. Failure of talc pleurodesis is associated with increased pleural fibrinolysis. Am. J. Respir. Crit. Care Med. 1995; 151 (3 Pt. L): 785-790.
43. Renne R., Brix A., Harkema J., Herbert R., Kittel
B., Lewis D., March T., Nagano K., Pino M., Ritting-hausen S., Rosenbruch M., Tellier P., Wohrmann T. Proliferative and nonproliferative lesions of the rat and mouse respiratory tract. Toxicol. Pathol. 2009; 37 (Suppl. 7): 5S-7S.
44. Strange C., Baumann M.H., Sahn S.A., Idell S. Effects of intrapleural heparin or urokinase on the extent of tetracycline-induced pleural disease. Am. J. Respir. Crit. Care Med. 1995; 151 (2 Pt. L): 508-515.
45.Tietze L., Bomtraeger J., Klosterhalfen B., Amo-Takyi B., Handt S., Gunther K., Merkelbach-Bruse S. Expression and function of pi and P3 integrins of human mesothelial cells in vitro. Exp. Mol. Pathol. 1999; 66 (2): 131-139.
46. Warn R., Harvey P., Warn A., Foley-Comer A., Heldin P., Versnel M., Arakaki N., Daikuhara Y., Laurent G.J., Herrick S.E., Mutsaers S.E. HGF / SF induces mesothelial cell migration and proliferation by autocrine and paracrine pathways.Exp. Cell. Res. 2001; 267 (2): 258-266.
47. Whitaker D., Papademitriou J. Mesothelial healing: morphological and kinetic investigations. J. Pathol. 1985; 145 (2): 159-175.
48. Whitaker D., Manning L.S., Robinson B.W.S., Shilkin K.B. The pathobiology of mesothelium. In: Henderson D. W., Shilkin K.B., Langlois S. L. P, Whitaker D., editors. Malignant Mesothelioma. New York: Hemisph. Pub.Corp .; 1992: pp. 25-56.
Received 15.02.2012
Contact information
Dmitry Acheksandrovt Semenov, Candidate of Medical Sciences, Senior Lecturer of the Department of Histology, Amur State Medical Academy, 675000, Blagoveshchensk, st. Gorky, 95.
E-mail: dimentii3 @ yandex.ru Correspondence should be addressed to Dmitriy S. Semenov, MD, PhD, Senior lecturer of Department of Histology>, Amur State Medical Academy, 95 Gor’kogo Str., Blagoveshchensk, 675000, Russian Federation.
E-mail: [email protected]
90,000 What is Mesothelium?
Mesothelium and related cancers, mesothelioma, are relatively unknown to most people.It is most common in men, but sometimes in women.
Mesothelium is a membrane that lines three body cavities: pleura or chest cavity; peritoneum or abdominal cavity; and the pericardium or heart sac. Mesothelial tissue is also found in the internal reproductive organs in men and covers the internal reproductive organs in women.
Mesothelium comes from the cell layer of the mesoderm, which lines the body cavity in its embryonic state. This becomes a layer of cells that covers and protects our internal organs.Mesothelium has two layers of cells. The first layer immediately surrounds the organ, and the second layer is the sac that surrounds the cavity.
Mesothelium produces a lubricating fluid that is released between the two layers, allowing moving organs such as the beating heart, dilated lungs, and active colon to glide easily into the body cavity.
Mesothelioma is a cancer in which cells in the mesothelium become abnormal and divide uncontrollably. They can invade and damage nearby tissues and organs, and cancer cells can also spread from their original location to other parts of the body.Most cases of mesothelioma begin in the abdomen. Mesothelioma is associated with exposure to asbestos and smoking.
Early symptoms of mesothelioma are usually nonspecific and can lead to a delay in diagnosis. Sometimes resembling viral pneumonia, mesothelioma patients who have lung disease may complain of shortness of breath, chest pain, or a persistent cough. Some patients have no symptoms at all. Symptoms of abdominal mesothelioma may include abdominal pain or swelling, weight loss, bowel obstruction, anemia, or swelling of the legs.
Remember that only a doctor can make an accurate diagnosis. Sometimes the symptoms of mesothelioma are very similar to less serious conditions, so be sure to discuss your symptoms with your doctor.
OTHER LANGUAGES
International Classification of Diseases (ICD-10) – D19.0
AGREEMENT ON TERMS OF USE OF INFORMATION POSTED ON WWW.WHITE-MEDICINE.COM
In accordance with national legislation, the information posted on this site can only be used by healthcare professionals and cannot be used by patients to make decisions about the use of these drugs.This information can not be considered as a recommendation for patients on the treatment of diseases and can not serve as a substitute for medical advice with a doctor in a medical institution. Nothing in this information should be construed as an appeal to non-specialists to independently purchase or use the described drugs. This information cannot be used to make a decision to change the order and mode of use of the drug recommended by the doctor.
No claims can be made to the site owner / publisher for any damage or harm suffered by a third party as a result of the use of published information that led to a violation of antitrust laws in pricing and marketing policy, as well as on regulatory compliance issues, signs of unfair competition and abuse of dominance, misdiagnosis and drug treatment of diseases, and misuse of the products described herein.Any claims of third parties on the reliability of the content, data provided by the results of clinical trials, compliance and compliance with the study design with standards, regulatory requirements and regulations, recognition of their compliance with the requirements of current legislation cannot be addressed.
Any claims regarding this information should be addressed to representatives of manufacturing companies and holders of registration certificates of the State Register of Medicines.
In accordance with the requirements of the Federal Law of July 27, 2006 N 152-FZ “On Personal Data”, by sending personal data through any forms of this site, the user confirms his consent to the processing of personal data within the framework, according to the regulations and conditions of the current national legislation …
.